Nitrogen+Syngas 366 Jul-Aug 2020
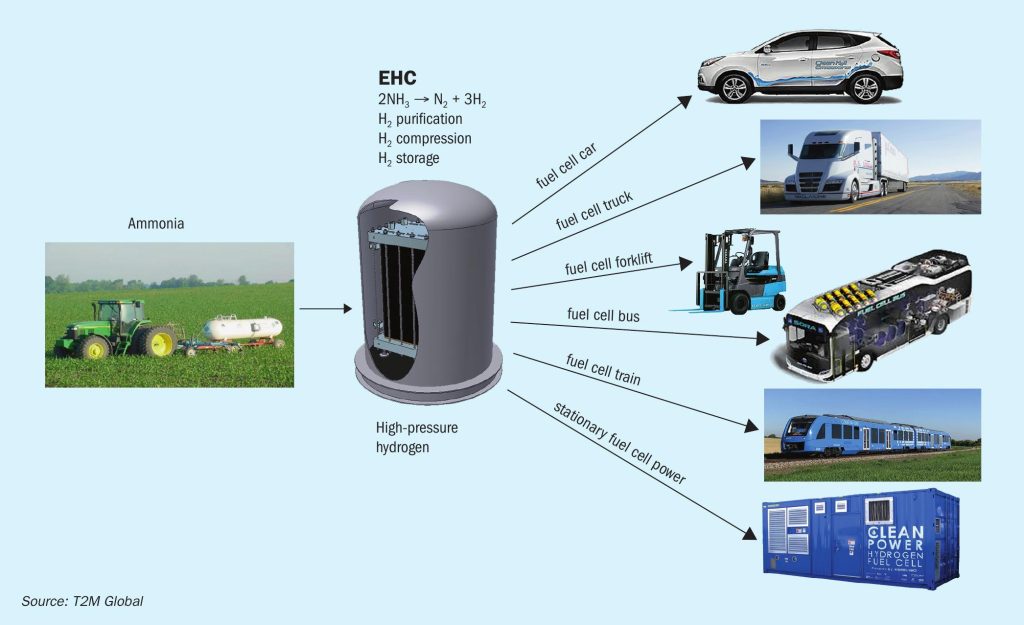
31 July 2020
Hydrogen for fuel cell vehicles and stationary power
AMMONIA TO HYDROGEN
Hydrogen for fuel cell vehicles and stationary power
As the world focuses on clean fuels for the 21st century, there is an emerging opportunity to use ammonia as a source of hydrogen. Hydrogen fuel cells are expected to play a significant role as part of a clean energy portfolio. T2M Global and its development partner, SAFCell, are collaborating to develop a modular system to produce hydrogen from ammonia using a novel solid state separation system that does not require pressure or solvents. P. Patel of T2M Global, C. Chisholm of SAFCell and V. Pattabathula of Incitec Pivot explain the challenges, the opportunities and the current status of this new technology.
The quest for clean energy for stationary and transportation sectors with zero emissions is now focusing on hydrogen fuel cell systems, which has become a multi-billion dollar industry worldwide. From kilowatt to multi-megawatt, fuel cell power plants are being deployed in USA, South Korea, Japan, and Europe. Fuel cell powered forklifts, cars, buses, trucks, ferries, and trains are becoming common. China is entering this rapidly emerging market in a very ambitious fashion with multibillion dollars of investments in fuel cell technology and hydrogen infrastructure. Hybrid engines coupling fuel cells with batteries have shown robust performance and enhanced economic viability. The cumulative market for hydrogen fuel cells has grown from MW/year to GW/year.
In parallel, government mandates and incentives for clean renewable energy have resulted in unprecedented growth in solar and wind installations worldwide. While highly beneficial, these renewables are highly intermittent. As their penetration increases, so does the imbalance between supply and demand in the electric grids. These intermittent renewables must be supplemented with synergistic and transportable energy sources. The emerging energy storage portfolio includes batteries for rapid response and hydrogen for long-duration response. Hydrogen as energy storage is particularly attractive for low-cost renewable energy. During peak output renewable energy can be converted on-site to hydrogen and stored as chemical fuel for transportation and stationary power applications. This distributed capability enables production cost reduction with minimal environmental impact. Hydrogen infrastructure has now become an important requirement to sustain growth in the clean and green energy arena.
Hydrogen infrastructure challenges
The unique advantage of fuel cells is distributed generation of clean electricity on-site and on-demand. This requires on-site hydrogen refuelling stations, typically about one tonne/day in capacity. These refuelling stations are expensive. The first challenge is that fuel cells require high-purity hydrogen for efficient performance (99.99+ %). Carbon monoxide must be below ppm level.
The second challenge is the cost of delivered hydrogen being too high. It has come down from a level of $20/kg to about $10/kg, but to be competitive, it must come down further to below $5/kg. This is a huge challenge for distributed users of hydrogen since they are not buying it in bulk (kg/day, not tonne/day). The low-density hydrogen is notorious for being hard to store. High pressure compressed hydrogen storage (5,000 to 10,000 psi) or liquid hydrogen storage is used to increase the capacity range of fuel cells in different applications (forklifts, cars, buses, or trucks). The hydride-type hydrogen storage has limitations of lower capacity, typically around 5% by weight, which limits their economic viability for fuel cell use.
On-site production of hydrogen using water electrolysis has been in use at some sites, with typically less than one tonne/ day production capacity. The operating cost of electrolysers is highly dependent on the cost of electricity and the amount of electricity needed per kg of hydrogen produced (50-60 kWh/kg hydrogen). This mode of hydrogen production cost is typically over $10/kg.
Ammonia as opportunity energy carrier
Ammonia offers a number of unique advantages as a source of hydrogen. It has an extensive distribution network worldwide, through pipelines, ships, trucks, and barges. All safety and user protocols are well established. It is a carbon-neutral liquid fuel and produces no GHG emissions. It produces no carbon monoxide, a serious advantage for fuel cell applications. Since there is no combustion in fuel cells, the nitrogen present in ammonia does not produce any harmful NOx emissions. It offers the highest energy density (~18 wt-%) among all other hydrogen carriers. Ammonia in liquid state (at room temperature and at reasonable 10 atm pressure) allows for easy transportation and cost-effective storage. Liquid ammonia can be easily compressed to desired high pressures with much lower energy consumption than gaseous hydrogen. Thus, ammonia is a highly promising carrier of hydrogen for fuel cells for both transportation and stationary power applications. At $300 per tonne of ammonia, it will contribute less than $2/ kg of hydrogen.
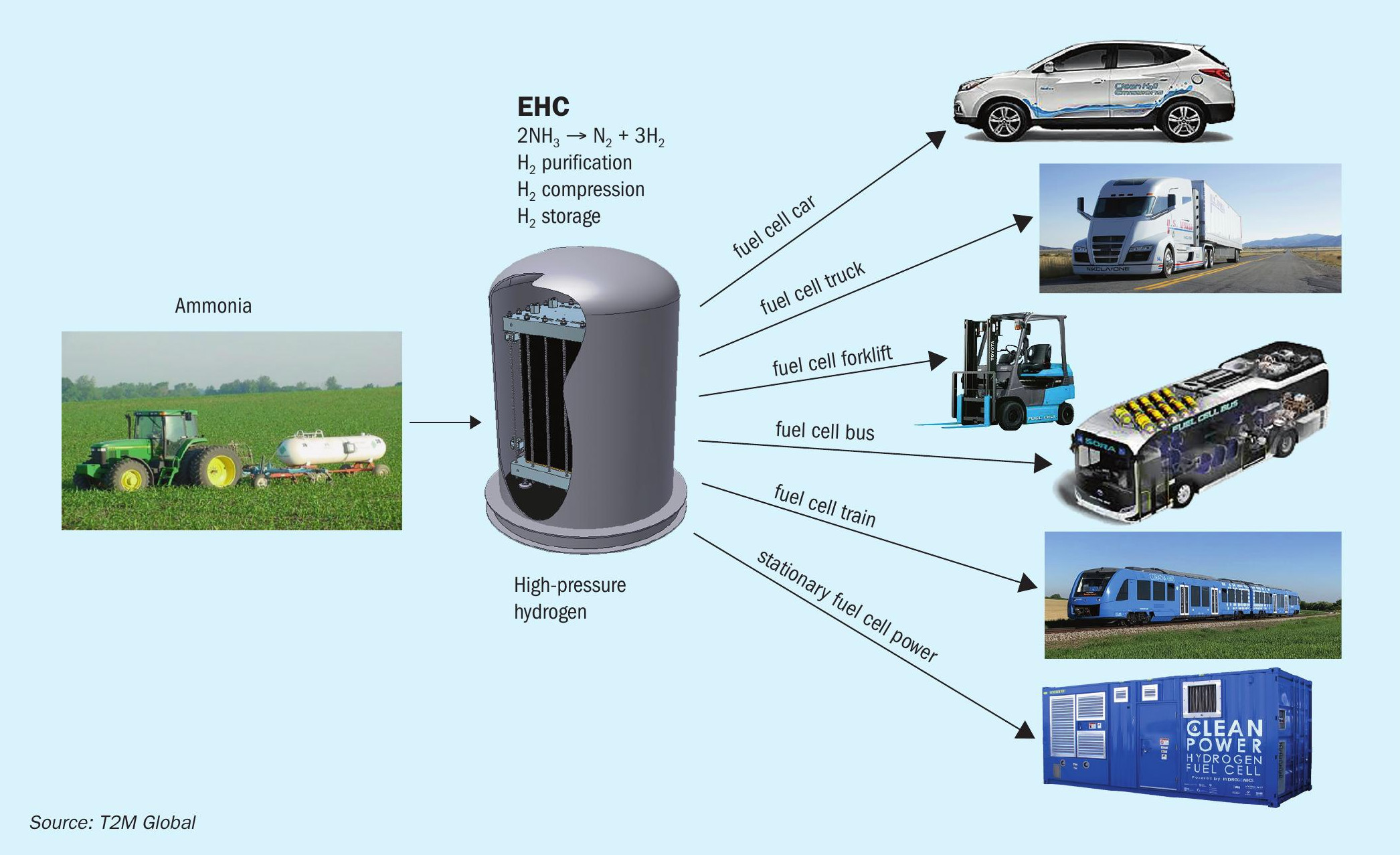
Ammonia opens a pathway to cost competitive hydrogen infrastructure for the multi-billion dollar emerging fuel cell industry. For one MW of fuel cell operating continuously at full load it is estimated that approximately 10 t/d ammonia will be needed. A fuel cell bus or truck (100 kW-class) will need up to 1 t/d ammonia. Anhydrous ammonia is already produced as a fertilizer and transported for agricultural use worldwide. It might already make economic sense in some areas to use some of the ammonia supply for producing hydrogen.
Technology development for ammonia to hydrogen
The first step is to crack ammonia into its components, hydrogen and nitrogen, followed by separating hydrogen from ammonia and nitrogen. Advanced materials such as membranes and catalysts and new electrochemical processes are required to efficiently generate hydrogen at higher conversion rates needed for competitive costs. Subsequent hydrogen purification processes like pressure swing adsorption (PSA) suffer from poor capacity to separate nitrogen cost-effectively. Under US Department of Energy (DOE-ARPA E) sponsorship, SAFCell, Inc. and T2M Global Team are developing advanced technology to extract hydrogen from ammonia in a single step using solid state electrochemical hydrogen separator and compressor (EHC). Fig. 1 shows different fuel cell applications that can be serviced by the EHC Module. It is highly scalable to service kW-class applications as well as MW-class with multiple modules. The EHC operating principle is illustrated in Fig. 2.
The electrochemical hydrogen compressor (EHC) is a single stage hydrogen separator and compressor. With catalyst embedded within the cell layer, it can crack ammonia internally, thus eliminating the need for an external cracker. The working principle is extremely streamlined as a result of this all-in-one feature (Fig. 2): A hydrogen carrier such as ammonia enters the stack (1). It cracks into hydrogen and the associated by-product internally (2). The catalyst advancement has enabled the cracking temperature to be reduced significantly, lowering the thermal energy requirements and maintenance costs associated with high temperature operation. Hydrogen ions are moved with >99.999% selectivity from one electrode (3) to another via a proton conducting membrane (4). The separated hydrogen ions form hydrogen gas on the catalyst layer (5). The product hydrogen is internally compressed to the desired pressure (30-100 bar) for direct on-demand fuel use.
Unique features and benefits
The key innovation is the use of a solid acid electrolyte as a proton conducting layer, a type of electrolyte that is stable in the presence of ammonia while under the operating conditions needed for reactions. Solid acid stacks operate at intermediate temperatures (around 250°C) and demonstrate high tolerance to typical anode catalyst poisons such as carbon monoxide and hydrogen sulphide without a significant decrease in performance. The system also aims to realise the conversion of ammonia along with the purification and compression of hydrogen in a single, cost-effective system, thus greatly simplifying the infrastructure required to transport and store hydrogen. These properties give solid acid EHC advantages over other membrane technologies in cost, durability, start/stop cycling, fuel flexibility, and simplified system design. If successful, EHC technology will be an enabler for the multi-billion dollar hydrogen fuel cell industry and create new markets for the ammonia industry, potentially doubling its capacity worldwide. It will enhance energy security and promote greater use of solar and wind energy for greater sustainability. It will create new higher paying jobs and enhance energy security.
Current status
Tremendous technology advancement has been made validating the EHC technology in single cells followed by scale up to multi-cell stacks. Stable stack operation at elevated pressures (up to 35 bar) has been successfully demonstrated for the first time ever using the solid acid membrane (Fig. 3).
EHC stack operation showed lower electricity consumption (<10 kWh/kg of hydrogen compared to 50+ kWh/kg of hydrogen for conventional water electrolysis). Ammonia cracking internally at lower temperatures (250°C) has been successfully demonstrated. In addition, a low-cost system design has been developed to recover and reuse the uncracked ammonia via patent pending R-3 technology.
Next step
Leveraging promising test results, technology scale up (single stack module for 40-100 cell stack with product hydrogen storage) is currently being conducted. This will be followed by prototype module design, fabrication, and testing (Target: 5 kg/day hydrogen from ammonia for home refuelling). The commercial system goal per US DOE is about 500 kg/day hydrogen (approx. 3 t/d ammonia).
Ammonia industry perspective
It is believed the newly developed low cost hydrogen from ammonia would increase the demand for ammonia production worldwide once H2 fuel cells are commercialised. With no GHG emissions when ammonia is cracked, many countries could move to ammonia as fuel as soon as the technology is available.
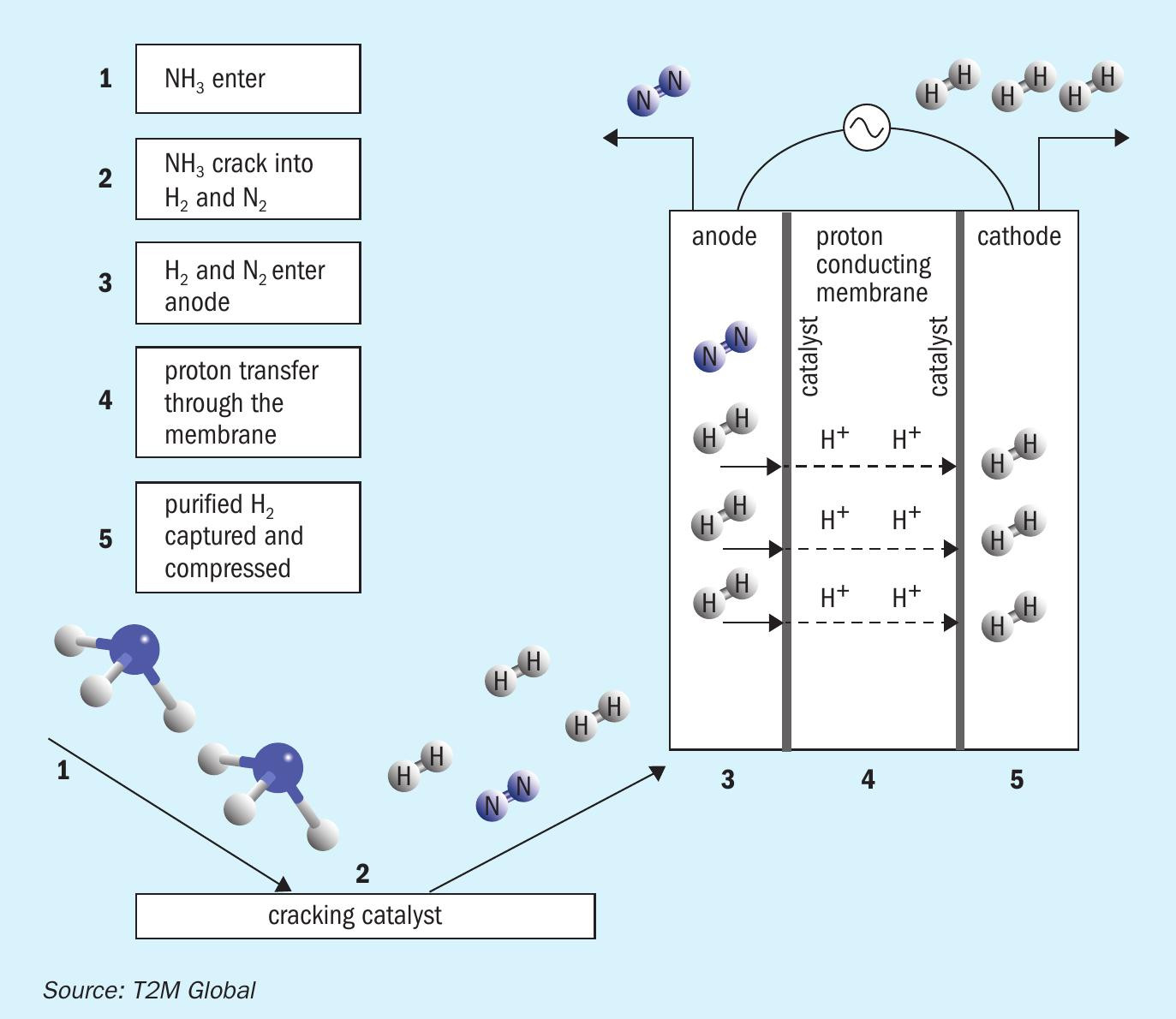
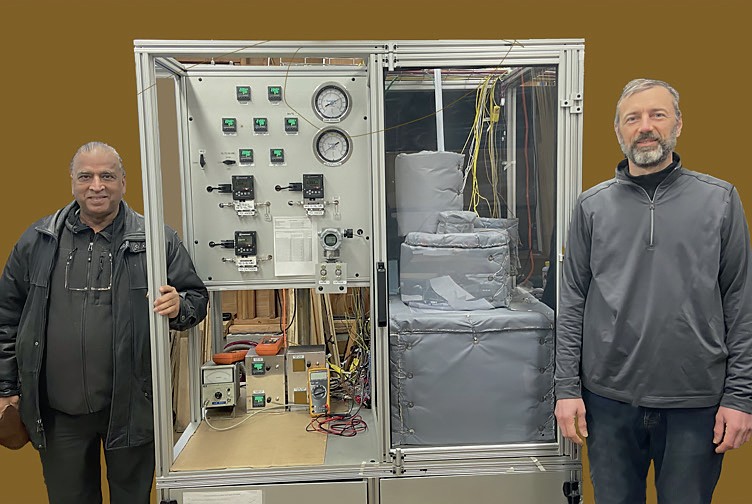
The development team could work with global fuel-cell and vehicle manufacturers to bring hydrogen fuel cell technologies to operate forklifts, buses, light & heavy vehicles, and ferries.
Acknowledgement: Financial support from US DOE is gratefully acknowledged. Contributions from Dr Ludwig Lipp, Ed Ko for EHC, test station work, and SAFCell members for ammonia and EHC cell-stack are recognised.