Nitrogen+Syngas 377 May-Jun 2022
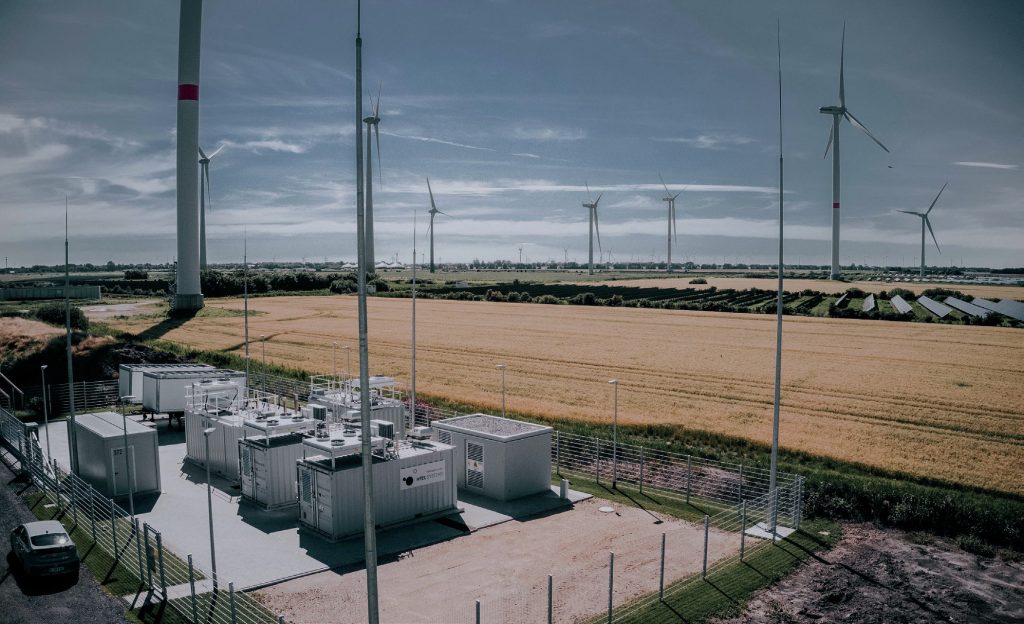
31 May 2022
The potentials of power-to-X and green fuels
GREEN HYDROGEN
The potentials of power-to-X and green fuels
Florian Gruschwitz of MAN Energy Solutions takes a look at the current investment decisions influencing green hydrogen projects on the path to decarbonisation, reviews technologies that are available today, and discusses what it will take to ramp up a global green hydrogen economy.
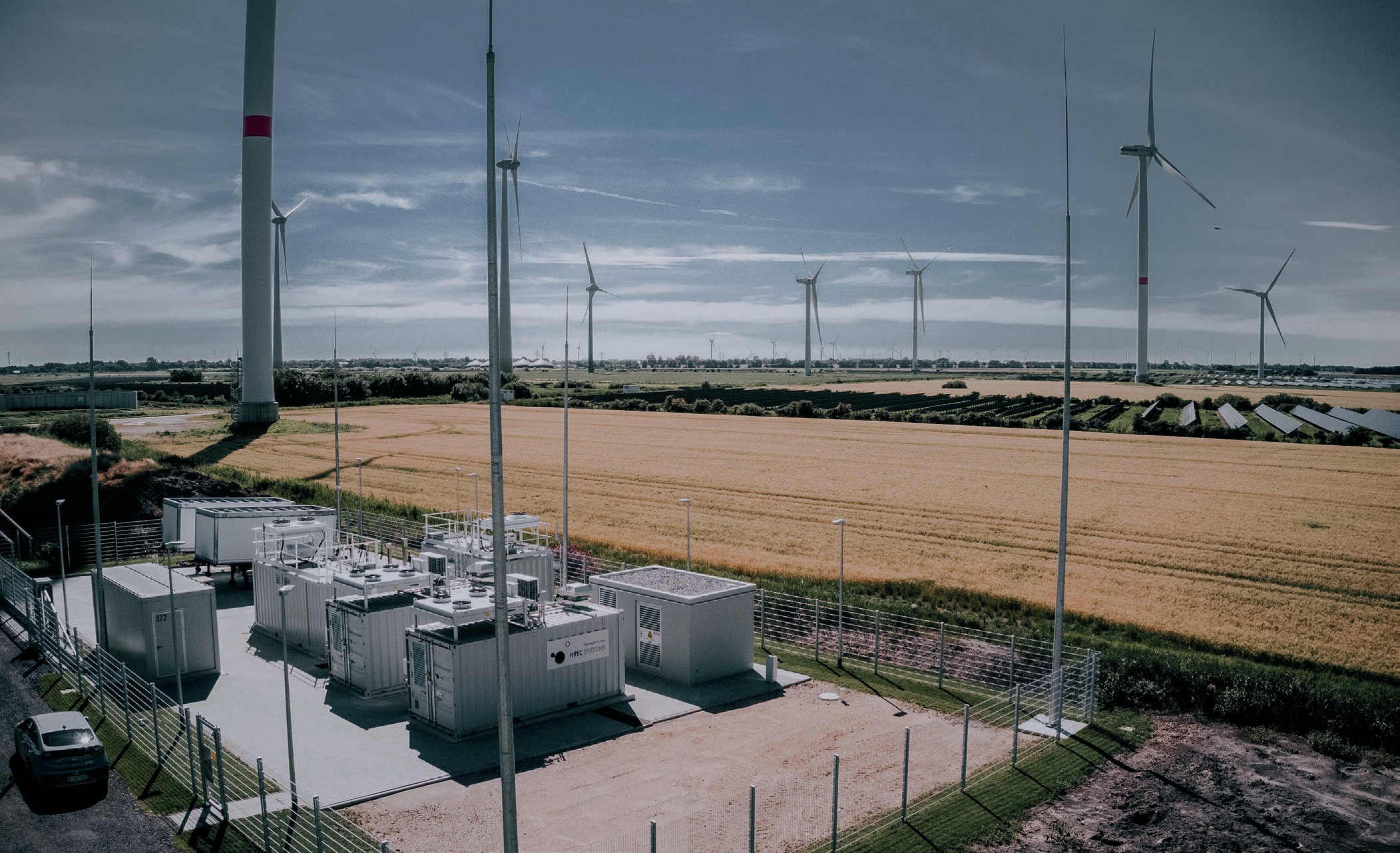
There is no doubt that green hydrogen is a key element on the path to decarbonisation. Nor is there even the least surprise these days that green hydrogen, and power-to-X in general, has gained so much popularity and public attention. For good reason, this will not be a flash in the pan.
Strong drivers like the EU’s ‘Fit-for-55’ programme, which targets reducing net greenhouse gas emissions by at least 55% by 2030,underline the reality that decarbonisation has now become a serious target and many countries have already published ambitious hydrogen strategies. Companies like MAN Energy Solutions can already provide the necessary key technologies along the power-to-X and green hydrogen value chain and have serious involvement through significant investments aimed at further extending the base of necessary technologies.
Mature technologies, for instance for e-fuel production, are available that enable the use of existing infrastructure, but much remains to be done in order to create more viable business cases. It can be shown how derivative fuels, or e-fuels, can successfully complement green hydrogen in its elemental form and be an important enabler in the ramp-up to a green hydrogen economy.
One thing is clear: elemental green hydrogen will not be a one-size-fits-all solution. Instead, there will be a multi-option scenario where pragmatic approaches will aim at maximum efficiency, whilst at the same time ensuring that a solid base and ramp-up path for long-term transition to green hydrogen is created (Fig. 2).
To get the full picture, it is helpful to look at the topic from two perspectives: firstly, viewing power-to-X in the context of how it can play an important role in reaching decarbonisation targets; and, secondly, looking at the main hurdles, but also success criteria, in getting a green hydrogen economy ramped up at a global level.
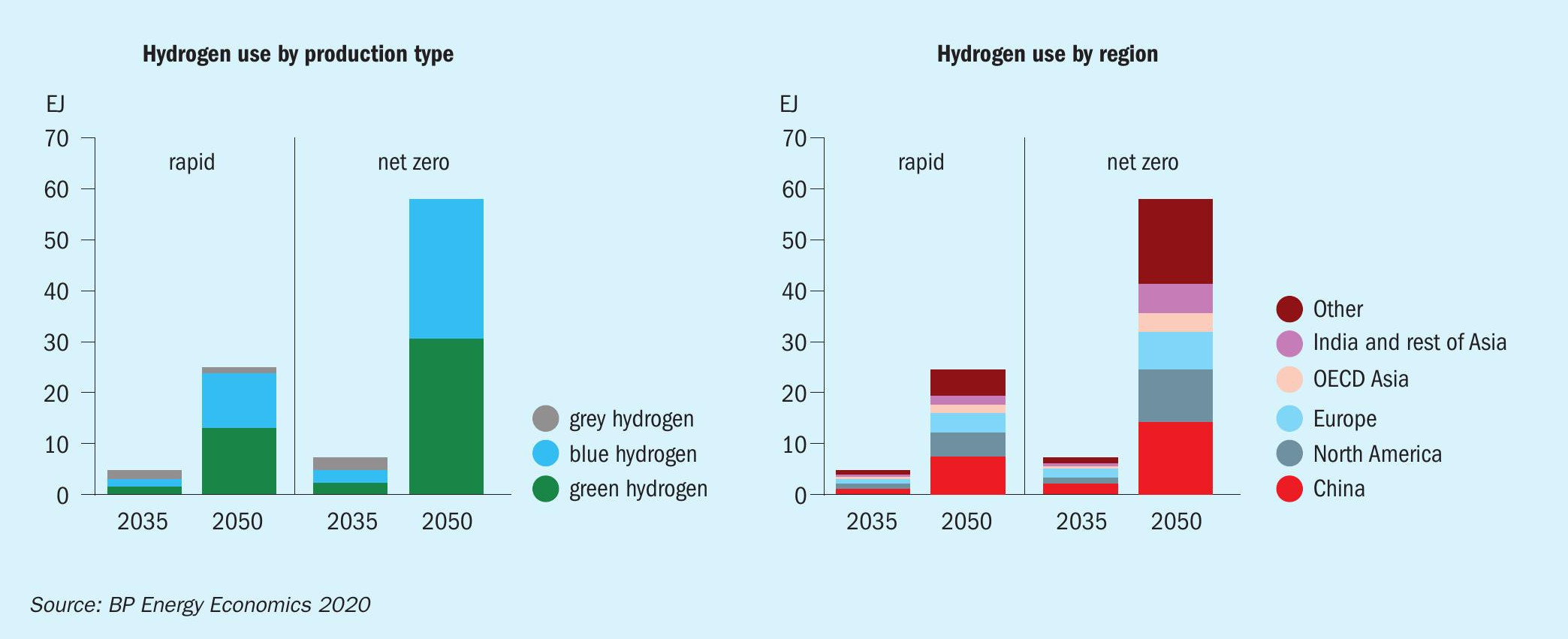
If we agree that decarbonisation is an underlying imperative in order to save the planet, then a policy comprising four elements can be identified, beginning with replacing fossil-fuelled power generation with renewable energy sources. The use of green hydrogen and employing e-fuels (based on green hydrogen) are two further elements. And the fourth, for the hard-toabate carbon sources, is carbon capture and storage technologies, again combined with power-to-X technologies.
These four elements may be viewed as a type of ‘decision tree’ such that, when addressing an application that acts as a considerable carbon source today, all four means of decarbonisation need to be assessed in the order shown to find the ‘best fit’ – i.e., the most effective way to achieve decarbonisation considering all current, boundary conditions.
Needless to say, decarbonisation is reliant upon an abundant availability of renewable energy. Accordingly, extending the capacity of renewable energy generation is of paramount importance. The first question in the quest for decarbonisation is therefore: ‘Is direct electrification possible?’. This means, first of all, replacing all fossil-fuelled power generation with renewable energy. However, natural-gas-fuelled power plants, for example, may be tolerated as ‘back-up’ or ‘peakers’ as they facilitate the maximum use of renewable energy in the grid while simultaneously ensuring maximum reliability and grid stability.
Continuing through the ‘decision tree’, for applications that cannot be directly electrified as of yet or even in the longer term, the use of green hydrogen could be a good option and many examples exist. However, following the Pareto principle, which specifies that 80% of consequences come from 20% of the causes, some prominent areas especially suited for decarbonisation can be identified, such as steel production where production with green hydrogen instead of coal would cut carbon emissions considerably.
Another good example of a sector ripe for decarbonisation with green hydrogen is within processes that already require large amounts of hydrogen today. Here, ‘grey’ hydrogen is currently used and produced by steam methane reforming. One such example is fertilizer production where ammonia as a main feedstock requires large amounts of hydrogen.
Which leads us to the third stage in the ‘decision tree’ when neither direct electrification nor the use of green hydrogen as a molecule is possible. In such instances, e-fuels may be a solution. Derivative fuels or e-fuels in this context are carbon-neutral fuels based on green hydrogen. This includes synthetic methane, methanol or ‘e-Kerosene’ – or ammonia produced from green instead of grey hydrogen, which provides a carbon-free option.
As such, derivative fuels could play an extremely important role: acting as a bridge technology and replacing their fossil twin, leading to carbon-neutrality; as a carrier medium for green hydrogen; or even as ‘green’ feedstock as for the prior-mentioned ‘green’ ammonia for fertilizer production. One of the great advantages in derivative fuels is their direct applicability today.
But even if we picture a fully electrified, green hydrogen and e-fuel-powered world, we must not forget that there are still applications or processes that intrinsically emit larger amounts of carbon. One very prominent example is cement production where, during the calcination process, large amounts of CO2 chemically bound within limestone are released. Pilot projects have already demonstrated, in order to reach the targeted ‘net zero’ for atmospheric emissions, that these carbon emissions can be captured, liquefied, and stored in subsea locations. Another method of reaching ‘net zero’ would be to use this CO2 to produce methanol as a chemical feedstock. In this way, carbon can be bound again as part of a cycle.
MAN power-to-X solution
MAN Energy Solutions is already a forerunner in power-to-X technology. In 2013, the company commissioned the methanation reactor for Europe’s first and for a long time most powerful power-to-gas plant on a 6 MW scale for Audi AG. Since then, MAN has consistently developed PtX technology and today offers turnkey plants with a capacity of 50 MW and more.
This MAN power-to-X solution is a sustainable solution for synthetic fuel production and long-term energy storage. It responds to the fundamental challenges of decarbonisation. The direct use of synthetic fuels allows the decarbonisation of sectors which currently rely on fossil fuels, such as marine, aviation or certain industrial processes.
Renewable energy is used to run an electrolyser, for example a PEM or an alkaline electrolyser, which breaks water down into hydrogen and oxygen. The hydrogen is then put into a methanation reactor with carbon dioxide, resulting in synthetic natural gas (SNG). The carbon dioxide can be obtained either by carbon capture from in-house or adjacent industrial processes or power generation using amine scrubbing, pressure swing absorption or membrane separation. The SNG can be stored, used directly, or injected into the existing gas infrastructure.
Hydrogen production by electrolysis
PEM electrolysis is a process by which electricity is used to split water into hydrogen and oxygen. It consists of a proton-permeable membrane, a cathode, and an anode. When water is added to the electrodes, the external voltage causes a catalytic effect, splitting the water. The hydrogen ions diffuse through the membrane.
To generate 1 kg of hydrogen, ~8.9 kg of water is required. In addition, ~7.9 kg of oxygen with a purity of 99.95% is produced. This corresponds to the purity required for further use in technical and medical applications. Water of tap water quality is required for electrolysis. The power requirement for 1 kg of green hydrogen is ~53 kWh.
H-TEC SYSTEMS is a subsidiary of MAN Energy Solutions and currently offers electrolysers with a nominal electrical output of up to 1 MW (Fig. 3). All H-TEC SYSTEMS solutions are integrated, scalable, and containerised. An electrolysis capacity of 1 MW provides enough hydrogen to fill a car tank up to 90 times per day in 24-hour operation. These module sizes are particularly suitable for pilot projects and small industrial customers. The electrolyser consists of 110 kW stacks, which can be replaced, if necessary, thus extending the service life of the plant. The maximum total electrolysis capacity is currently 10 MW but will be expanded to 150 MW in the future with the new product “Hydrogen Cube Systems” (HCS). These are 2-MW modules which make it possible to cater to applications with a high hydrogen demand.
H-TEC SYSTEMS electrolysers have an integrated water treatment and deionisation system. Therefore, only water that meets industrial standards (tap water) is necessary as a feedstock for electrolysis. In arid areas, additional water generation may be necessary e.g., with desalination plants.
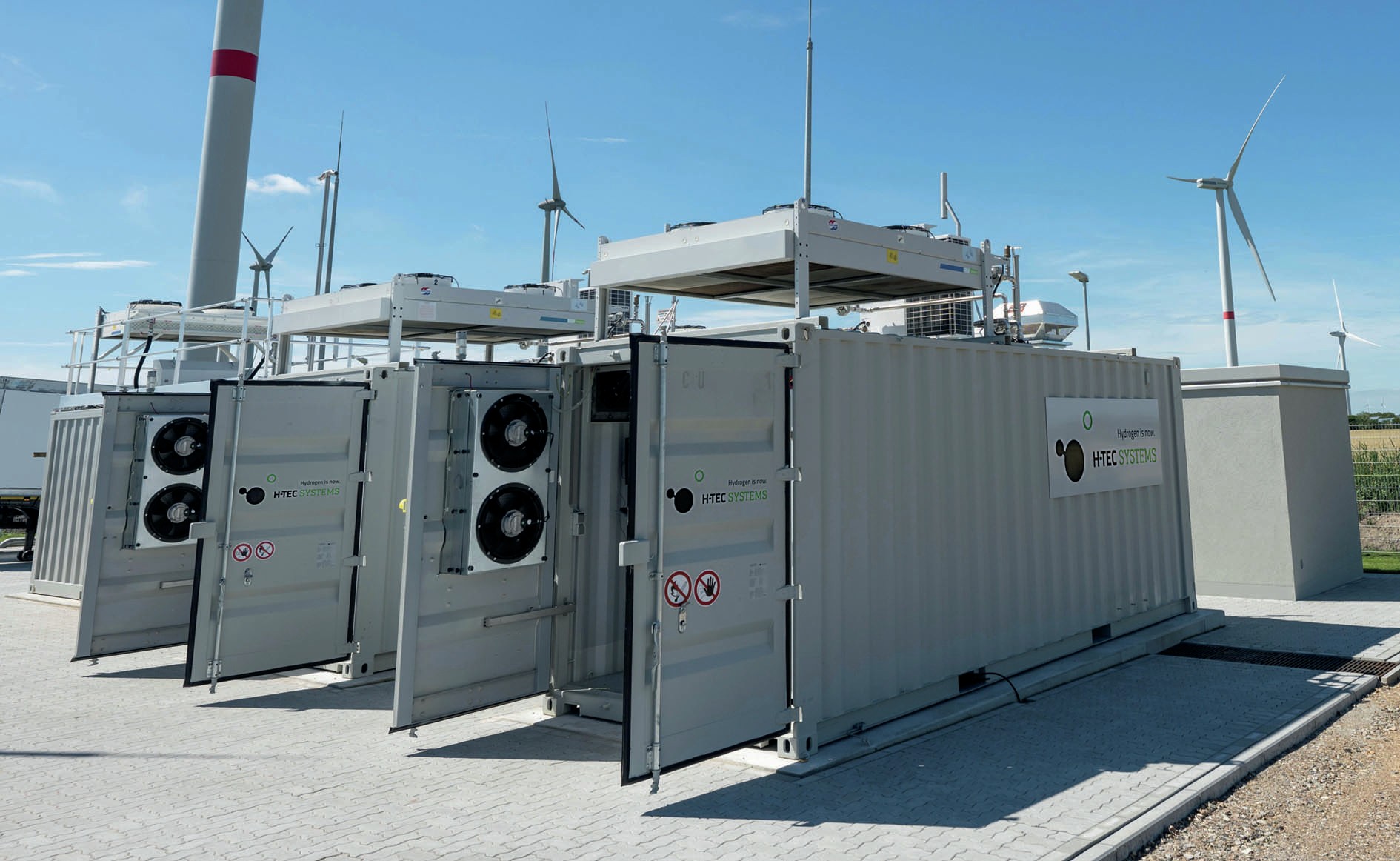
SNG production by methanation
Methanation, or the Sabatier process, is a chemical reaction in which carbon dioxide is converted to synthetic methane. It is an exothermic reaction that has to be accelerated by nickel catalysts. The chemical efficiency is ~83%.
From 1 kg of H2 , ~2 kg of SNG and ~4.5 kg of water are produced with the addition of CO2 . This is a chemical reaction that takes place without additional energy in the form of electricity. The equipment associated with the methanation reactor, such as pumps, requires electricity, so a total of ~27.3 kWh is required to produce 1 kg of SNG.
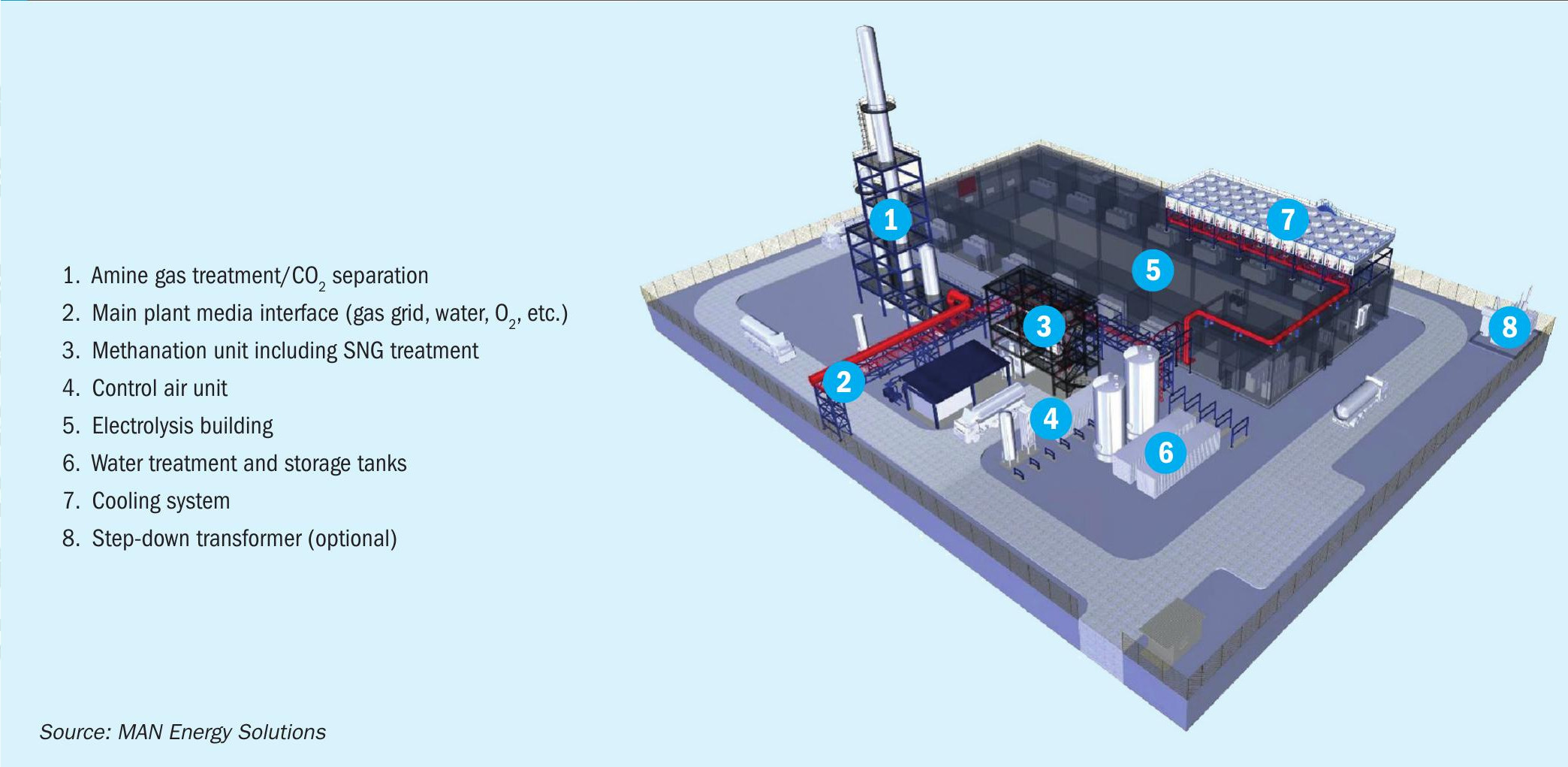
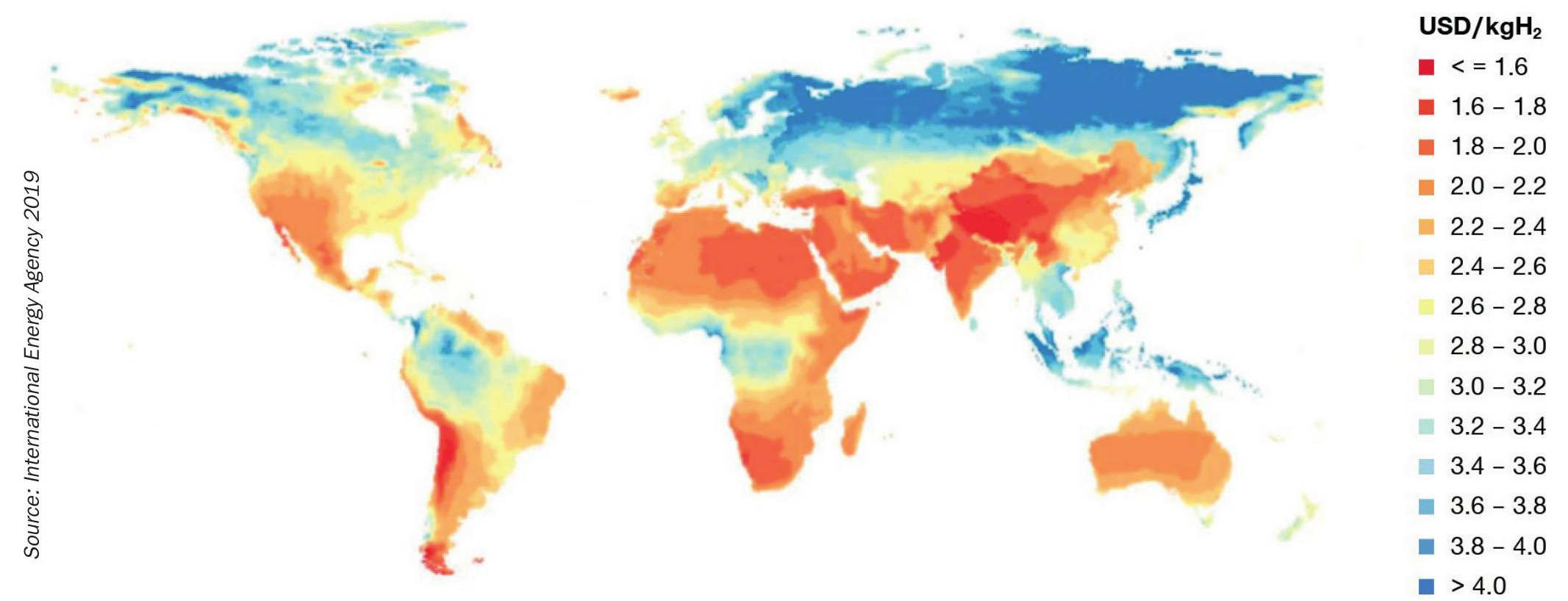
The reactor is a boiling water reactor in which process temperatures range from 270-600°C. The process by-products are water and saturated steam at a temperature of 270°C. If this is integrated into other production processes, the methanation process can achieve an overall efficiency of 95%. The outlet pressure is 20 bar(g).
Two reaction stages are necessary for high methane purity (>95%) in MAN methanation technology. This is due to the thermodynamic equilibrium that occurs between the two reaction sides. Water is separated in an intermediate stage between the two reaction stages. This means that, in the second stage, the equilibrium is shifted further to the product side and thus the highest gas purity can be achieved.
Since this is an exothermic reaction, external cooling is necessary to allow the chemical process to proceed in a controlled manner. There is no risk of damage to the equipment due to excessive temperatures. In addition, cooling influences the thermodynamic equilibrium in a positive manner and thus contributes to the final high product purity. Continuous cooling increases efficiency (a greater mass of reactants can be converted into products), requiring a smaller reactor and less catalyst material. The overall system is more compact than adiabatic process concepts, which require a total of three to five process steps with intermediate cooling to achieve a methane purity of >95%.
Fig. 4 shows a model of a complete 50 MW power-to-gas plant by MAN Energy Solutions.
The challenges
In conclusion, a carbon-neutral world, the desired “net zero”, to avoid further climate change is within reach and without having to completely change the world, the products we use, nor our way of life. Green hydrogen and power-to-X are key elements in this transition. The question then is: how do we ramp up the green hydrogen economy? For this, we will have to consider the whole value chain: the production of green hydrogen and derivatives, its transport to its application, and of course the application itself where, as in the case of direct reduction ovens for ‘green steel’ production, some considerable investments will be needed.
Accordingly, all parts of the value chain need to be pushed and ramped up simultaneously. Large, industry-wide programmes like Germany’s ‘H2 Giga’ initiative are helping to scale up electrolysis to industrial levels with accompanying cost-reductions. However, the cost reduction of green hydrogen production alone does not make for a feasible business case when green fuels have to compete with their fossil twin without integrating the external cost of additional carbon introduced to the atmosphere. Thus, respective carbon taxation is needed as well as, at least for the ramp-up phase, smart ‘Carbon Contracts for Difference’ schemes like the German ‘H2 Global’ to finally make larger power-to-X projects bankable.
Fig. 5 shows hydrogen costs from hybrid solar PV and onshore wind systems in the long term.
Setting up a global hydrogen economy is necessary to leverage renewable-energy potential in regions where it cannot be otherwise used and in order to not cannibalise renewable energy capacities in regions with high demand. This would also help to bring sustainable prosperity to more parts of the world and could solve strong global (inter)dependencies in energy trading.
Large-scale off-takers such as steel production have to be created, for example, in line with EU Important Projects of Common European Interest (IPCEI) projects. Even if they had to rely on ‘blue hydrogen’ in a starting phase, this means that investments could be made and hydrogen pipeline infrastructures created. Subsequently, as soon as green hydrogen production was at scale, a ‘switch’ to green hydrogen would be possible with all the major investments made up to that point in time. As such, it’s acceptable for many of the first, large power-to-X projects to rely on derivative fuels since ocean transport of elemental hydrogen is a challenge. E-fuels can complement a green hydrogen economy, are an enabler for larger electrolyser plant setups, and can resolve the chicken or egg dilemma until hydrogen grids become available to provide inexpensive transport, storage, and distribution options.
Seen from an industry perspective, we can say that we are ready and eager to shape the future. We are taking the risk and investing in the transformation of our portfolios and to provide the necessary technologies. Now we need the necessary political action in order to ramp up a global green hydrogen economy and to convert decarbonisation targets into reality.