Sulphur 405 Mar-Apr 2023
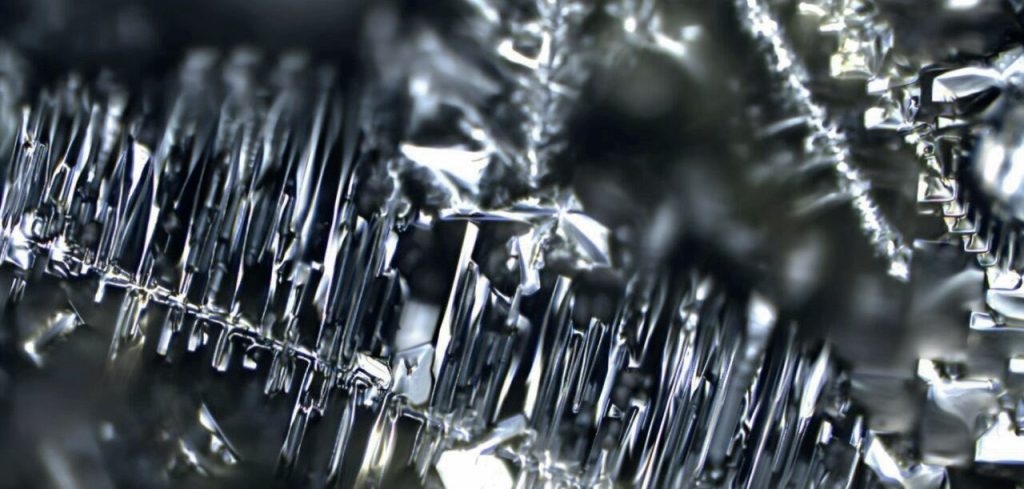
31 March 2023
Advances in sulphur battery technology
SULPHUR MARKETS
Advances in sulphur battery technology
Lithium sulphur batteries have many advantages over conventional lithium ion batteries in terms of energy density, and they also avoid the use of costly cobalt, but they deteriorate rapidly and cannot be recharged as frequently. However, recent advances in Li-S batteries could overcome this problem and lead to widespread adoption.
The electrification of our society, now beginning to extend into personal transportation as well as all of the other myriad ways we consume electricity these days, puts a premium on electricity storage, especially for mobile applications such as smartphones, laptops, tablets etc. More advanced mobile devices, with wi-fi connections streaming large files such as movies, place greater demands upon batteries, resulting in shorter battery lifetimes between charges. Electric vehicle performance is similarly limited in terms of vehicle range by their batteries’ capacity, and providing power via conventional lead-acid cells imposes a significant weight penalty.
Battery technology has been increasing steadily over the past few decades, moving to progressively higher energy densities in terms of Watt-hours per kilogram. A conventional lead acid cell can produce around 25-45 Wh/kg. The nickel-cadmium batteries that were developed in the 1940s expanded this to 40-60 Wh/kg, and in the 1990s nickel metal hydride batteries reached 75 Wh/kg. But undoubtedly the greatest breakthrough was the development of lithium-ion batteries, which can store up to 250 Wh/kg, and which have made the development of the present generation of personal electronics possible.
Lithium-based batteries now represent 40% of the global battery market, with the rapid increase in electric vehicles meaning that the use of Li-ion batteries is still growing at around 15% year on year. Even so, while it has allowed manufacturers to make your laptop, tablet or smartphone smaller and lighter, few of us are, I imagine, particularly impressed with the battery life we get between charges; usually only a few hours of continuous use. And while lithium itself is light, lithium-ion batteries typically require bulky cathodes, often made from ceramic oxides like cobalt oxide, to house the ions, which increases the weight and so limits the battery’s energy density.
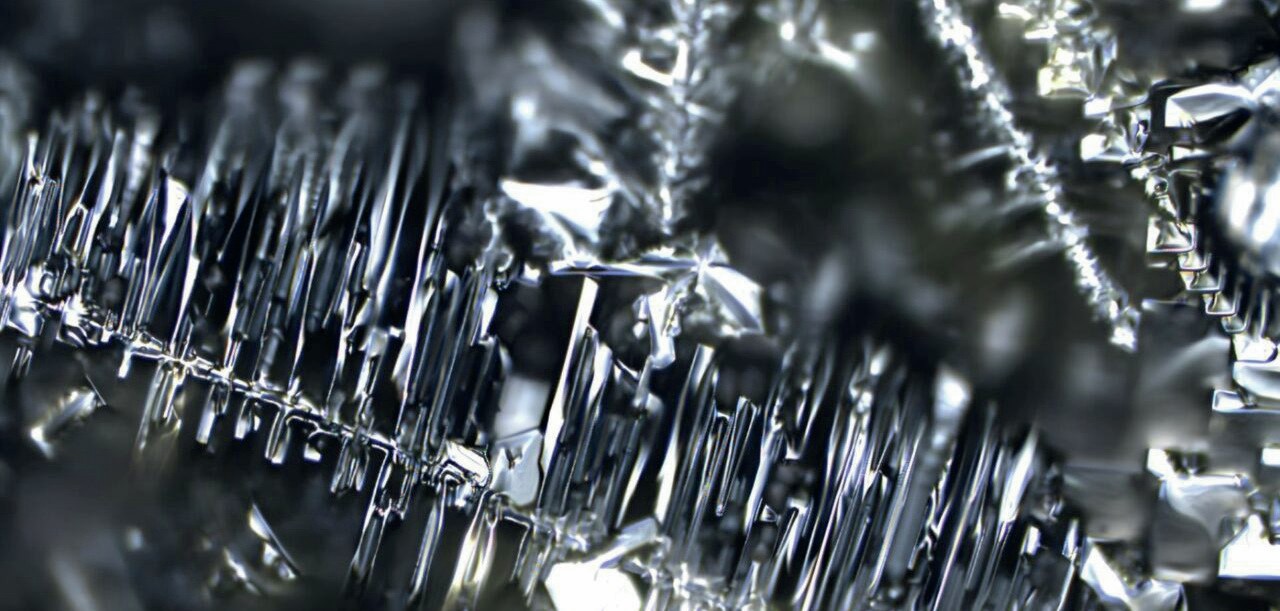
Using sulphur as a cathode, however, lowers the battery’s weight considerably, as well as increasing the energy density. Lithium-sulphur batteries can already achieve energy densities of 500 Wh/kg; twice that of lithium-ion batteries. This is the equivalent of doubling the range of an electric vehicle or the continuous usage time of a laptop or smartphone. And the theoretical limit of the technology is as high as 2,500 Wh/kg.
Technical issues
In theory, then, we should be switching to lithium sulphur batteries. However, the battery chemistry also presents some problems which have prevented its large scale commercialisation. Lithium and sulphur can form a wide variety of lithium polysulphide compounds with differing oxidation states, from Li2 S through Li2 S2 , Li2 S3 , Li2 S4 etc, all the way up to Li2 S8 . These form at the cathode as lithium migrates from the anode. However, higher lithium polysulphides tend to be soluble in the electrolyte, and over time this can destroy the structure of the cathode, leading to the formation of dendrite structures of polysulphide and cracks in the sulphur cathode with repeated cycling, reducing the number of times that the battery can be recharged.
Overcoming this issue has been the key to the development of Li-S batteries, and there are signs that progress is being made. Initially efforts focused on inserting a redox-inactive layer between the cathode and anode. But this layer was heavy and dense, reducing energy storage capacity per kg for the battery. More recently, researchers at the US Argonne National Laboratory have developed and tested a porous sulphur-containing interlayer. Tests showed initial capacity about three times higher in batteries with this active, as opposed to inactive, layer. More impressively, the cells with the active layer maintained this high capacity over 700 charge-discharge cycles; a performance similar to lithium-ion batteries.
Research also continues elsewhere. In Europe, the EU has been part funding the LISA (LIthium sulphur for SAfe road applications) project with 13 project partners throughout the EU and UK. Running since 2020, LISA identified nine promising lines of research for achieving their goals of a stable 450Wh/kg automotive LiS battery with 1,000 recharge cycles, and in 2022 the project moved to pilot production for these, for testing and validation in vehicle trials. Likewise there is considerable excitement in the US over the new Inflation Reduction Act (IRA) which offers large government subsidies for battery development.
Commercialisation
But perhaps the most promising recent development has been the announcement by Korean giant LG, through its energy arm LG Energy Solution, which supplies batteries for Tesla, amongst others, that it plans to try to commercialise a lithium-sulfur battery in 2025, with mass production by 2027. LG is also looking at aviation applications for the technology, with electric or electric-hybrid light aircraft using the new lightweight battery technology. In 2020, LG supplied Li-S batteries to an experimental unmanned drone aircraft, the EAV-3, developed by the Korea Aerospace Research Institute. The plane charged the batteries using solar power during the day and ran off battery power at night during a 13 hour test flight at an altitude of 12-22 km. Reportedly, the LNG battery uses a sulphur-carbon composite for the anode and lithium metal for the cathode. LG has been working with the Korea Advanced Institute of Science and Technology (KAIST), and announced in January 2023 that its research team, led by professor Lee Jin-woo has developed an energy density- and duration-improved lithium-sulphur battery using an iron cathode composite that reduces lithium polysulphide dissolution, albeit at the expense of some weight (the battery has a claimed energy density of 320 Wh/kg – still more then 50% higher than Li-ion).
Germany company Theion also claims to have developed a lithium sulphur battery, using crystalline sulphur with carbon nanotubes and a proprietary solid electrolyte. Theion grows a pure sulphur wafer by a ‘direct crystal imprinting’ method directly from molten sulphur. Because of the flexibility of the process, the company says that it can grow any geometrical shape of wafer adapted to a customer’s product shape. The company says it will be shipping its first batteries later this year, as with LG first to aerospace customers, as part of the qualification stage, and then aircraft, air taxis, drones, mobile phones and laptops, before servicing the electric flight and automotive sectors in 2024.
However, there have also been a number of exits from Li-S battery development. British tech startup Oxis, originally one of the partners in the LISA programme, went bankrupt in May 2021. Sion Power, which also claimed to be developing a Li-S battery, has moved onto lithium oxides instead.
Sodium sulphur batteries
Lithium sulphur batteries are not the only game in town. There is also the sodium sulphur battery, originally developed by Ford in the 1960s and subsequently sold to the Japanese company NGK. NGK now manufactures the battery systems for stationary power applications; the batteries use molten sulphur as the cathode and molten sodium as the anode, separated by a solid ceramic, sodium alumina, which also serves as the electrolyte. The ceramic allows only positively charged sodium-ions to pass through. The electrons that are stripped off the sodium metal move through the circuit and then back into the battery at the positive electrode, where they are taken up by the molten sulphur to form sodium polysulphide. The positively charged sodium-ions moving into the positive electrode compartment balance the electron charge flow. However, as well as the additional weight (sodium is heavier than lithium, and the alumina also adds to the weight), because it works in the liquid phase the battery must also be kept hot (typically >300°C) to facilitate the process, making it unsuitable for non-stationary applications. Nevertheless, the batteries are very efficient (up to 90%), and Na-S battery technology has been used at over 190 sites in Japan, with more than 270 MW of stored energy suitable for 6 hours of daily peak shaving installed. In Abu Dhabi, fifteen Na-S systems acting in coordination provide 108 MW / 648 MWh to defer fossil generation investment and provide frequency response and voltage control services. The market for Ns-S batteries is estimated at $480 million/ year at present, and is forecast to grow at 15% year on year as projects powered by intermittent renewable energy look to battery storage to even out the flow of electricity.
Lithium constraints
The cost and availability of sulphur is not a factor for Li-S batteries, indeed, it is one of their major selling points – it is less than 1% of the cost of the cobalt used in some other modern batteries. Lithium is a different story, however, and may be the key constraint in the production of Li-S batteries. In fact, concerns about the availability of lithium have already been raised in the context of lithium-ion batteries. Demand for lithium is expected to rise to 300,000 t/a by 2025 and more than 1.0 million t/a by 2030 because of lithium’s use in lithium ion and other batteries.
While this is a large increase in demand to meet, production of lithium is nevertheless increasing very rapidly. In 2021 total global output of lithium reached 106,000 t/a, four times its value a decade earlier, with batteries accounting for 75% of all lithium demand. Production is concentrated in just three countries; Australia, Chile and China represented 90% of production that year, but there are a number of new lithium projects across the world, particularly in the United States. Even so, lithium availability may put a cap on how fast lithium battery production is able to expand over the coming decade.
The impact on sulphur
With promising new developments in improving the battery technology, the commercialisation of lithium sulphur batteries is increasingly looking like a question more of ‘when’ rather than ‘if’. If LG are to be believed, then large scale production could be just a couple of years away. Once Li-S batteries are commercially available, and assuming that the technology works as advertised, it is quite possible that their uptake may be as fast as that for Li-ion, which cornered 80% of the market for personal electronics and vehicles inside a decade.
The actual volumes of sulphur required for Li-S battery production are unlikely to be large within the current decade; it may be a matter of a few hundred thousand tonnes per year at most by 2030. However, it may be the impact on sulphuric acid demand for lithium mining that has the largest effect. In the US, new lithium projects are expected to add 2 million t/a of additional sulphuric acid demand in the next couple of years, and if the boom extends elsewhere, this could mean several million tonnes of acid and a concomitant increase in sulphur demand.