Fertilizer International 521 Jul-Aug 2024
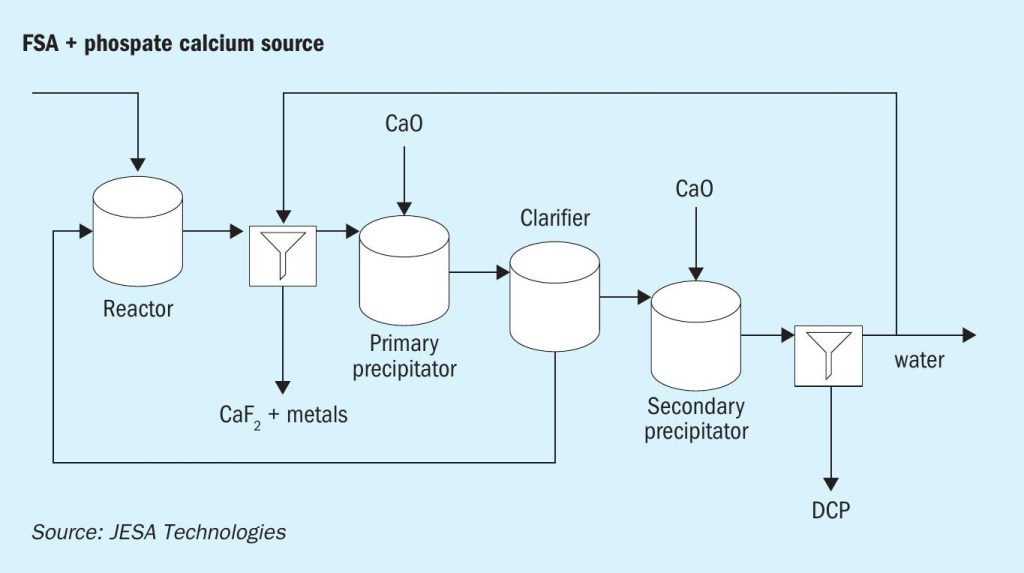
31 July 2024
SWIFT – a fluorine management game changer?
PHOSPHATE TECHNOLOGY
SWIFT – a fluorine management game changer?
The newly-patented SWIFT process is designed to sequester fluorine at phosphoric acid plants in an environmentally responsible way. It can also offset the costs of fluorine management by generating a saleable dicalcium phosphate (DCP) end-product as an additional revenue stream. A number of capex and opex advantages provide the SWIFT process with highly favourable economics, as James Byrd of JESA Technologies explains.
Introduction
JESA Technologies (JT) launched SWIFT, a new fluorine management technology for phosphoric acid plants, at CRU’s Phosphate 2024 event in Warsaw earlier this year (Fertilizer International 520, p48). While primarily designed to sequester fluorine in an environmentally responsible way, the SWIFT process can also offset the costs of fluorine management by providing additional revenues. It does this by generating dicalcium phosphate (DCP) as a commercially saleable product. The flexibility of SWIFT is a key advantage as it means the process can be adapted and applied differently to match local circumstances.
Background
Fluorine is a common constituent of phosphate deposits globally and is therefore typically present, to a greater or lesser extent, in the phosphate rock feed consumed at phosphoric acid plants.
Fluorine emissions during phosphoric acid production are becoming an increasingly important Environmental, Social and Corporate Governance (ESG) concern for both fertilizer producers and investors. Controlling these emissions effectively is problematic, however, due to the limited range of fluorine management options available to operators currently.
During phosphoric acid production, fluorine is dissolved during the acidulation of phosphate rock concentrate and, once in solution, can escape as vapour at key points in the process. Vapour pressure, and therefore emissions, are a function of phosphoric acid concentration, system pressure and fluid temperature.
Precisely where fluorine emissions will emerge at a given production plant is determined by the phosphoric acid process technology. Fluorine emissions from dihydrate (DH) plants, for example, are greater during the concentration stage at the back end of the plant, while hemihydrate (HH) plants emit more fluorine during the reaction and filtration stages at the plant’s front end.
The primary way to capture and control fluorine at phosphoric acid plants is by recovering as much fluorosilicic acid (FSA) as possible. Fluorine emissions are converted to FSA by transferring these to the liquid phase, usually in barometric condensers or scrubbers, in the presence of adequate silica and water. FSA recovery units can be incorporated in the original plant design. Recovery can also be implemented at existing plants through brownfield projects designed to capture fugitive emissions, although this does require careful evaluation of the recycle and washing chemistry.
What to do with the FSA produced remains a major question though. Historically, it has been necessary to neutralise FSA or convert it into anhydrous hydrofluoric acid (AHF) or aluminium fluoride (AlF3 ) as the commercial market for FSA is very limited and low value. Yet all of these options still present difficulties. The production of AlF3 involves outdated technology, for example, while AHF production involves significant capex and usually requires vertical integration with downstream processes as transporting AHF is potentially hazardous. The other remaining option, typically the neutralisation of FSA with lime, is also an expensive high opex process.
In our view, the new SWIFT process, in contrast to the costly alternatives described above, can help solve the fluorine removal riddle, both responsibly and economically, while also producing dicalcium phosphate (DCP) as a valuable by-product.
Process description
The starting point for the SWIFT concept is the PECO process originally developed by the Phosphate Engineering & Construction Co in Florida in the late 1980s, although it differs in purpose and incorporates major refinements. The PECO process was designed to produce both relatively pure phosphoric acid and a saleable fluorspar as a precipitate. The process, which involves reacting FSA with phosphate rock, was run by one commercial phosphate producer for almost a year. But the phosphoric acid generated was weak and of lower quality, compared to purified phosphoric acid (PPA), while the fluorspar obtained was unable to compete with commercial-grades on the open market as it was less pure.
The basic SWIFT process involves four main steps (Figure 1). A phosphate source is initially acidulated with FSA in a reactor. The filtrate obtained is transferred to a primary precipitator and the pH adjusted with quicklime. This results in the formation of fluorspar, which comes close to molar completion, and the precipitation of monocalcium phosphate (MCP). This pH adjusted mixture is then pumped to a clarifier.
Following clarification, the solids are pumped back to the reactor, where MCP is digested and enters solution, while the fluorspar remains as a solid under the reaction conditions. The filtrate from the clarifier undergoes another pH adjustment before entering a secondary precipitator. This generates DCP as a solid and relatively clean water as a filtrate. The latter can be reused within the SWIFT plant or sent to the phosphoric acid plant for use as make-up water in the fume scrubber or cooling tower. DCP can be generated in either anhydrous or dihydrate form by adjusting the pH target.
This is a simplified process description and SWIFT can be altered in response to the actual reaction chemistry and/or the end purpose. In contrast to the PECO process, the fluorspar obtained is classed as waste and is disposed of, possibly with gypsum at the phosphoric acid plant. The presence of impurities within the fluorspar is therefore not a concern and, in fact, may even be beneficial to overall plant operations.
Key advantages
The key advantages of the SWIFT process are its ability to:
- Produce DCP for different purposes
- Accept different and lower grade phosphate feedstocks
- Be applied to any phosphoric acid plant technology
- Offer an attractive net present value (NPV) for both greenfield and brownfield plants.
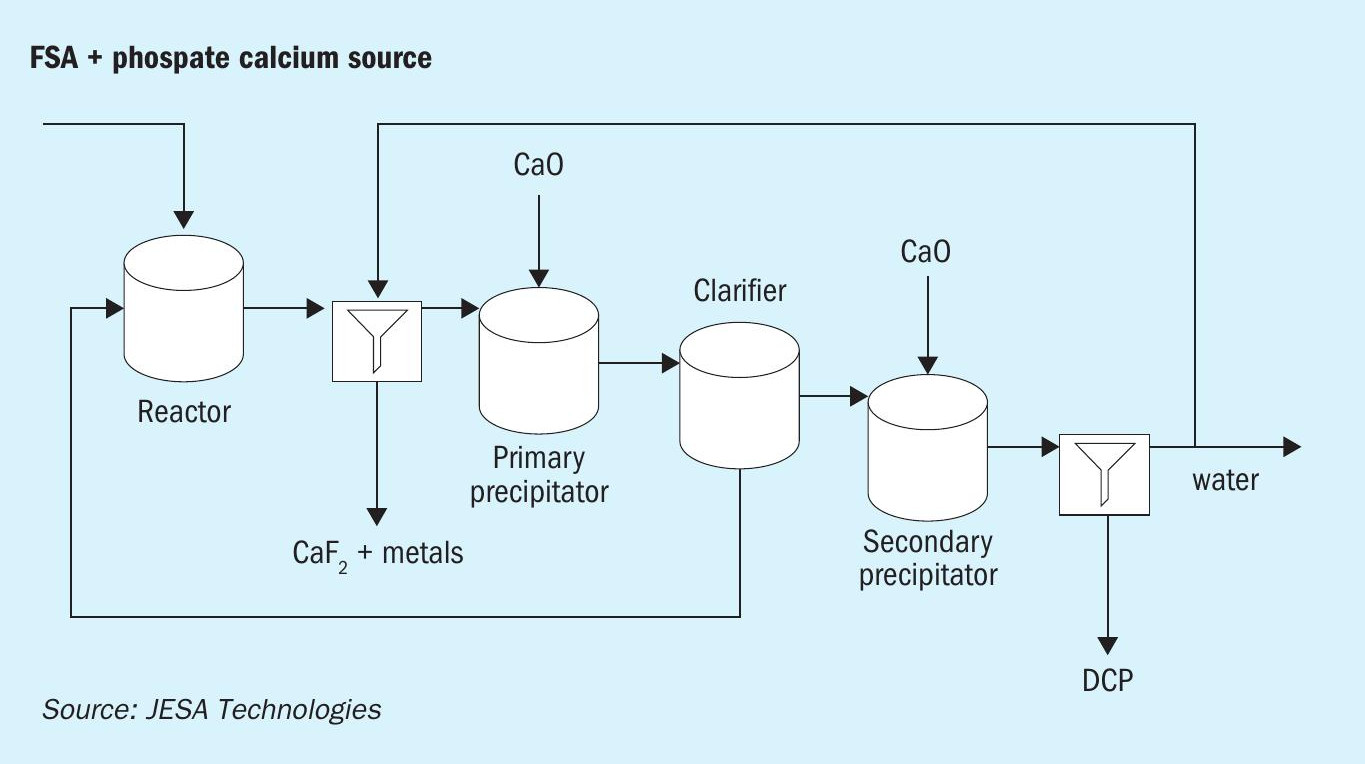
SWIFT has the potential to produce a relatively pure and saleable DCP product.
There is more to the process, though, than simply selling DCP on the open market.
Indeed, if the SWIFT process was implemented at phosphoric acid plants globally, it is conceivable that the merchant DCP market could become oversupplied with prices depressed as a result. Fortunately, there are three proven and viable end-use options for DCP:
- Sales on the commercial market
- Integrated use as feed for a phosphoric acid plant producing merchant grade acid (MGA)
- Integrated use as feed for a phosphoric acid plant producing purified phosphoric acid (PPA).
Valuably, because SWIFT is a flexible process, its final flow sheet can be configured differently for each of these three end-uses.
SWIFT offers other advantages too. For example, unlike the PECO process, SWIFT does not require beneficiated ore (phosphate rock concentrate) as a feedstock. Instead, less costly P 2 O 5 sources are perfectly acceptable. These include beneficiation wastes (e.g., tailings or coarse rejects) or the lowest incremental cost run-of-mine (ROM) ore. These sources can improve phosphoric acid plant project economics as the P 2 O 5 from the beneficiation wastes is generally written off – with any extra P 2 O 5 recovery providing an economic bonus.
The SWIFT process can be used with any phosphoric acid plant (PAP) technology. In fact, SWIFT can be applied to any industry process, including those completely unrelated to fertilizer production, where fluorine is solubilised and concentrated.
SWIFT’s flexibility also means it can be applied to both greenfield (new) and brownfield (existing) plants – although the opportunities for achieving benefits and synergies are more straightforward in greenfield plant design. While brownfield projects can also deliver benefits, the potential synergies generally need more careful study. Typically, by installing SWIFT for fluorine management, fluorine scrubbing will be more efficient and, consequently, total fluorine recovery should increase with a corresponding decrease in fugitive emissions.
In the dihydrate (DH) phosphoric acid process, FSA is normally produced from the flash chamber in the evaporator as this is where the acid vapour pressure is exploited. FSA is generated using a specifically-designed scrubbing vessel that washes vapours until the concentration of FSA in the recirculated water reaches the desired target. More than 90 percent of the fluorine present in the vapour phase can be recovered in a well-designed FSA scrubber. This generates good quality FSA with low impurity levels at a concentration of between 22-24 percent.
In contrast, incorporating the SWIFT process at a greenfield DH plant will allow a much smaller FSA scrubber unit to be installed – because the FSA target concentration is closer to five percent rather than 22-24 percent. Furthermore, the entrainment separator can be omitted as P 2 O 5 contamination is not a concern. These design changes can deliver significant capex savings, while the extra P 2 O 5 carried through will generate more DCP. Also, because any fluorine-laden stream can be recovered in the SWIFT process, blowdown from the fume scrubber can also be used to generate FSA.
While the above example is for the DH process, SWIFT can be used with any phosphoric acid plant technology.
SWIFT has a similar capex to a traditional FSA neutralisation unit. The reactor does not require internal heating as the FSA can be heated externally as it is being pumped into the reactor. The steam condensate collected in phosphoric acid plant evaporators can be used for this purpose. It makes an ideal heat source, given that the SWIFT reactor is relatively small with a relatively short residence time.
For the SWIFT process, the lime consumption of the primary and secondary precipitator is also a fraction of that consumed by a traditional neutralisation plant, as both precipitators are also small with short residence times. This means SWIFT can delivers an opex saving. Associated equipment can also to be downsized as well. The two filters used in the process (Figure 1) are smaller compared to the filters in the phosphoric acid plant, for example, as the flow volumes are lower.
These capex and opex advantages, when combined with the benefits of DCP production, favour the economics of the SWIFT process in a big way.
DCP can be used as feed for the phosphoric acid plant (PAP) reactor. This can be a favoured option if the merchant DCP market is oversupplied, or when the phosphate ore has high levels of impurities. The substitution of relatively pure DCP for phosphate rock decreases the minor element ratio (MER) of the feed to the reactor. This helps improve yields, production rates and product quality at both the PAP and fertilizer granulation plant.
Another benefit of using DCP as a reactor feed is lower sulphuric acid consumption due to its reduced calcium to P2 O5 ratio. Correspondingly, production output and filtration rates will also increase due to lower impurity levels. Steam consumption in the evaporators can even be reduced if the P2 O5 concentration of the phosphoric acid is raised. Less gypsum should also be generated thereby reducing waste handling costs.
The final end-use option for DCP is as feed for purified phosphoric acid (PPA) plants. This is likely to be an increasingly attractive option in the future as PPA production could double in size over the next 20 years to supply the growing market for lithium iron phosphate (LFP) batteries used in electric vehicles (Fertilizer International 517, p46). The acidulation of DCP to generate PPA is a well known process with a long history in the phosphate industry. Advantageously, a DCP-fed PPA plant would have fewer unit operations than a traditional PPA plant and would also produce pure gypsum.
Conclusion
Regardless of the reasons for implementing the SWIFT process, this new technology upholds ESG goals by providing operators with a more sustainable production process that delivers economic benefits. SWIFT’s flexibility offers operators a variety of end-use options that can meet site-specific goals and can also deliver potentially valuable synergies at phosphate fertilizer complexes. Consequently, the valorisation of fluorine has never been this affordable, in our view, with far reaching environmental and economic benefits.