Fertilizer International 501 Mar- Apr 2021
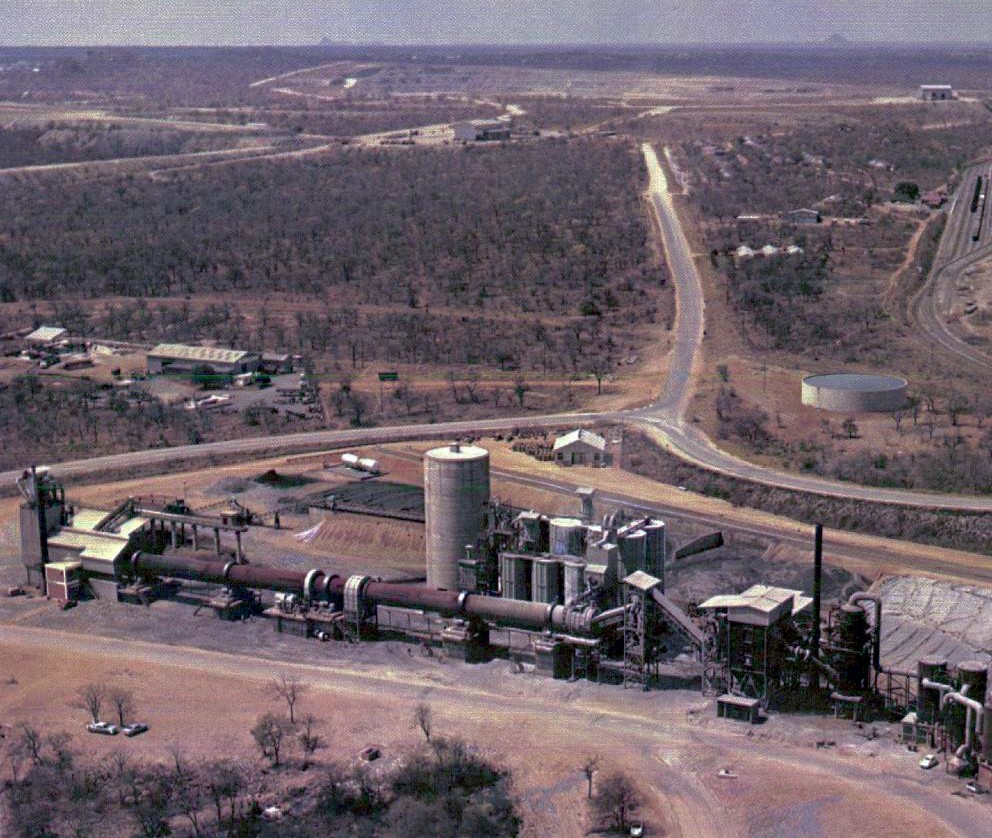
31 March 2021
Phosphogypsum: joining the circular economy?
USING PHOSPHOGYPSUM
Phosphogypsum: joining the circular economy?
The economic conversion of phosphogypsum waste into a valuable product has been pursued for decades. Results of intensive research by thyssenkrupp Industrial Solutions (tkIS) in this area are presented by Peter Stockhoff, Dirk Koester, Stefan Helmle and Carsten Fabian. The approach developed by tkIS shows great potential as a controlled treatment process for phosphogypsum.
Large volumes of phosphogypsum (PG) are generated during the production of phosphoric acid from natural phosphate rock using sulphuric acid. About 4-6 tonnes of PG are generated as a waste by-product for every tonne of phosphoric acid produced. This corresponds to annual PG production of around 250 million tonnes globally – roughly equivalent in weight to 1.25 million family houses.
Although gypsum is the main constituent of PG, a range of other impurities can also be present, including residual phosphate, fluoride, organic matter, iron, aluminium, heavy metals and radionuclides (Fertilizer International 478, p58). Certain countries have placed legal restrictions on the disposal and use of PG due to the presence of these impurities.
PG is often stored indefinitely above ground as a wet slurry in engineered containments known as ‘stacks’. Stockpiling in wet stacks is common industry practice. More than one billion tonnes of PG is stacked in Florida, for example, the main centre of US phosphate production. Large phosphate operations may need to open one or more PG stacks during their lifetime (Fertilizer International 478, p58).
PG stacks typically occupy large areas of land near phosphoric acid plants. They are usually designed and operated according to strict regulations, making them costly to construct and maintain. As a consequence, their safe management is a major cost burden and environmental challenge for phosphate plant operators.
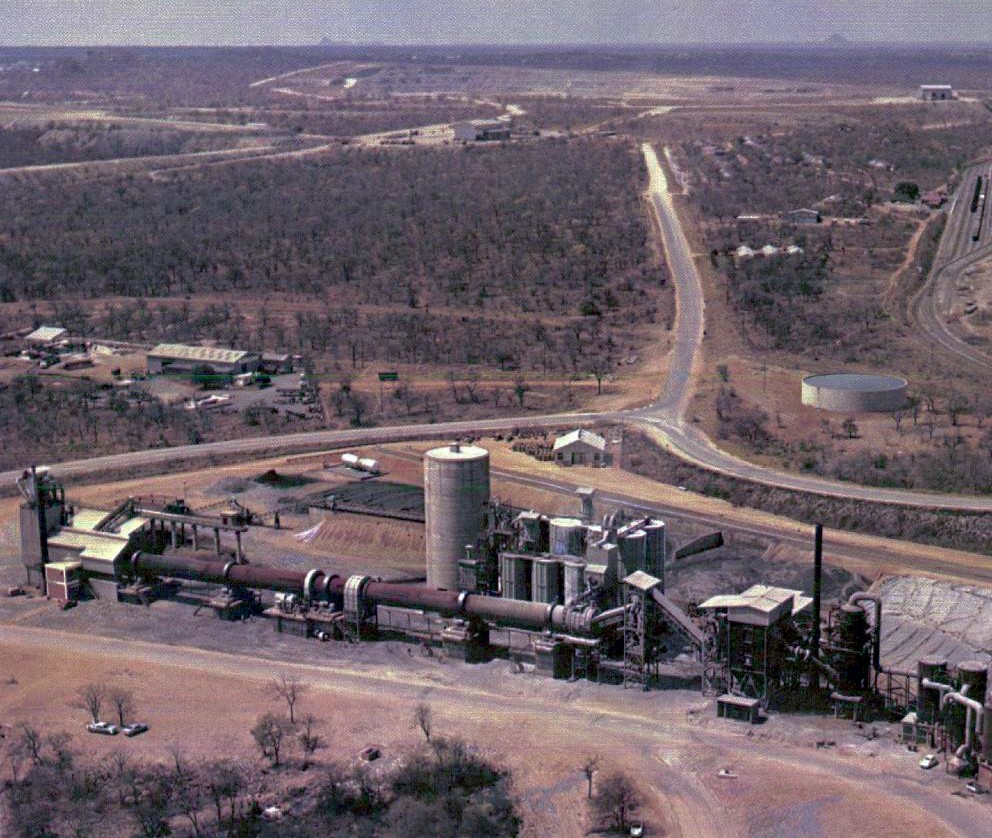
Although industry attitudes are changing fast, PG is still generally disposed of and stored as waste. Currently, just 15 percent of the vast amounts of PG generated globally goes on to be used in some form. Nevertheless, common end-uses include:
- In agricultural as a fertilizer
- Land reclamation
- Soil remediation and conditioning
- Building materials – particularly the cement and plaster market
- Road construction.
The OSW-Krupp process
The OSW-Krupp phosphogypsum process was responsible for some promising early examples of commercial PG use. This process combined phosphogypsum with additional clay, sand, coke and natural gypsum to generate equal amounts of sulphuric acid and Portland cement.
A number of OSW-Krupp plants were built between 1956 and 1980 in South Africa, Poland, Austria and Germany. Although their main purpose was to recover sulphuric acid from PG via thermal decomposition, valuable clinker for the cement industry was also produced.Cement clinker is an intermediary in the manufacture of Portland cement, and is conventionally produced by sintering limestone and clay in a cement kiln.
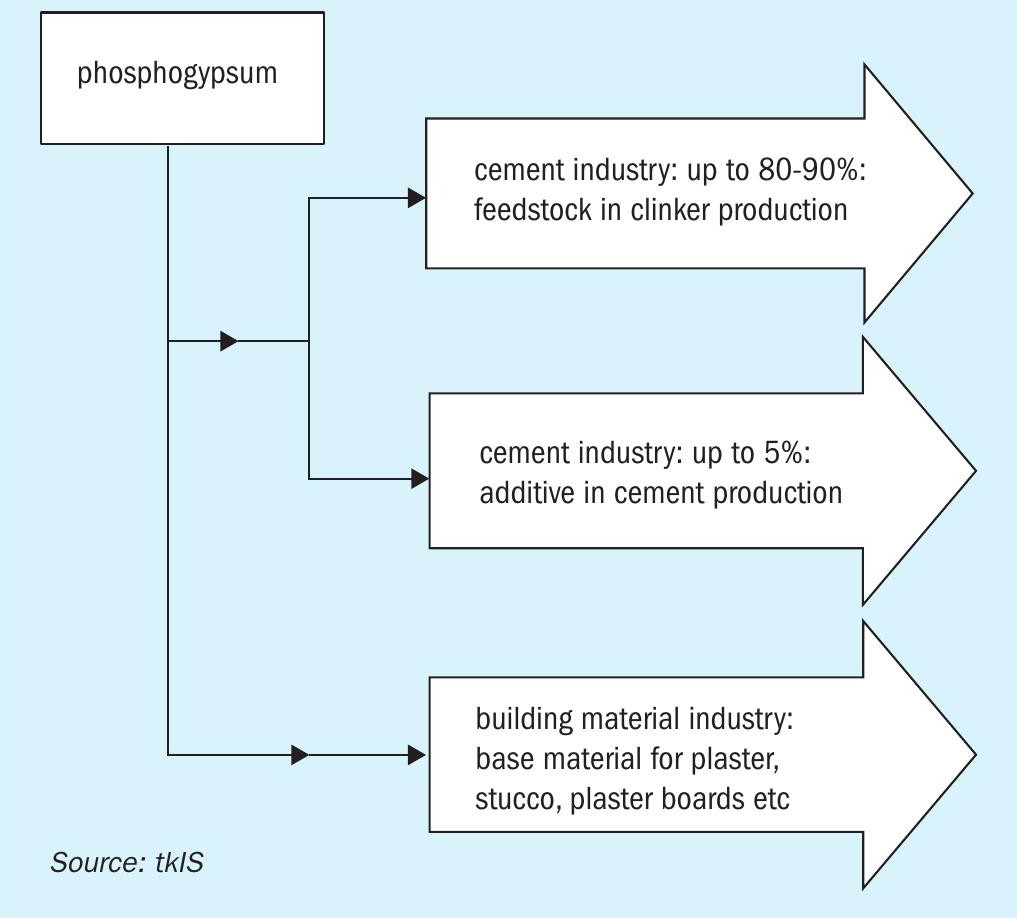
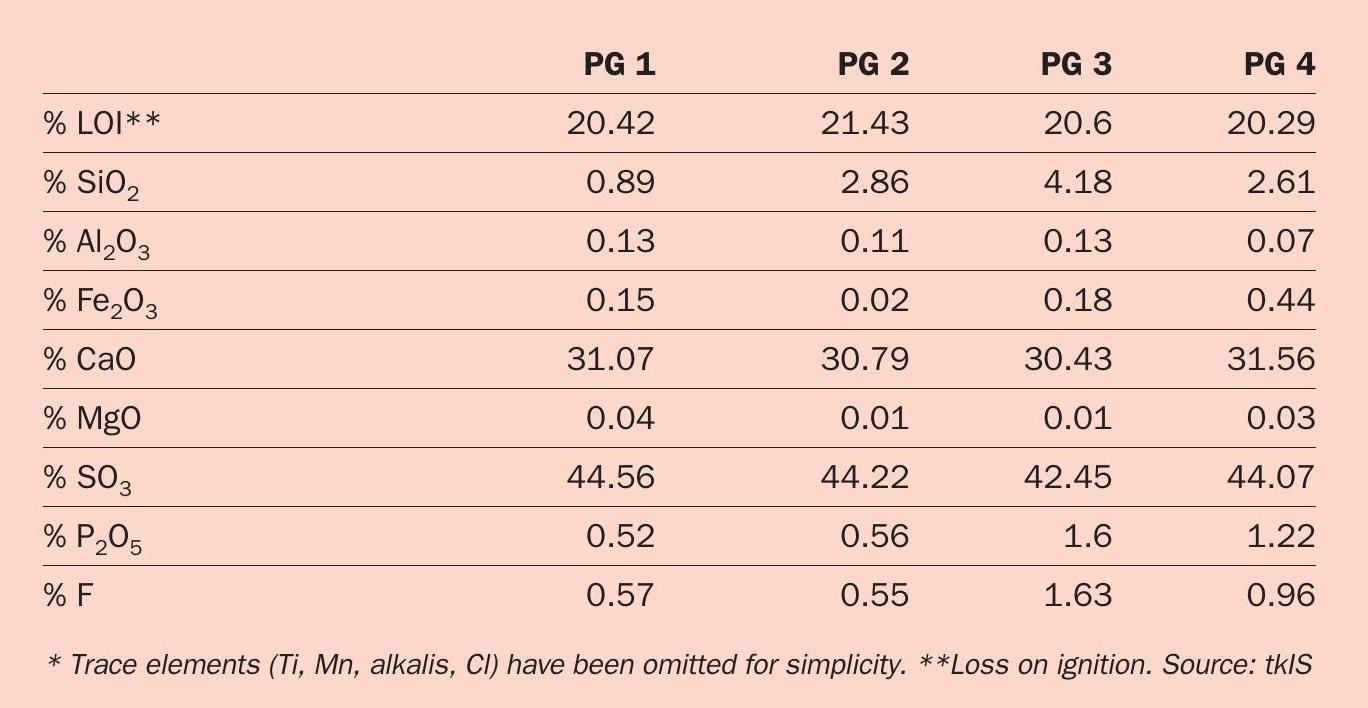
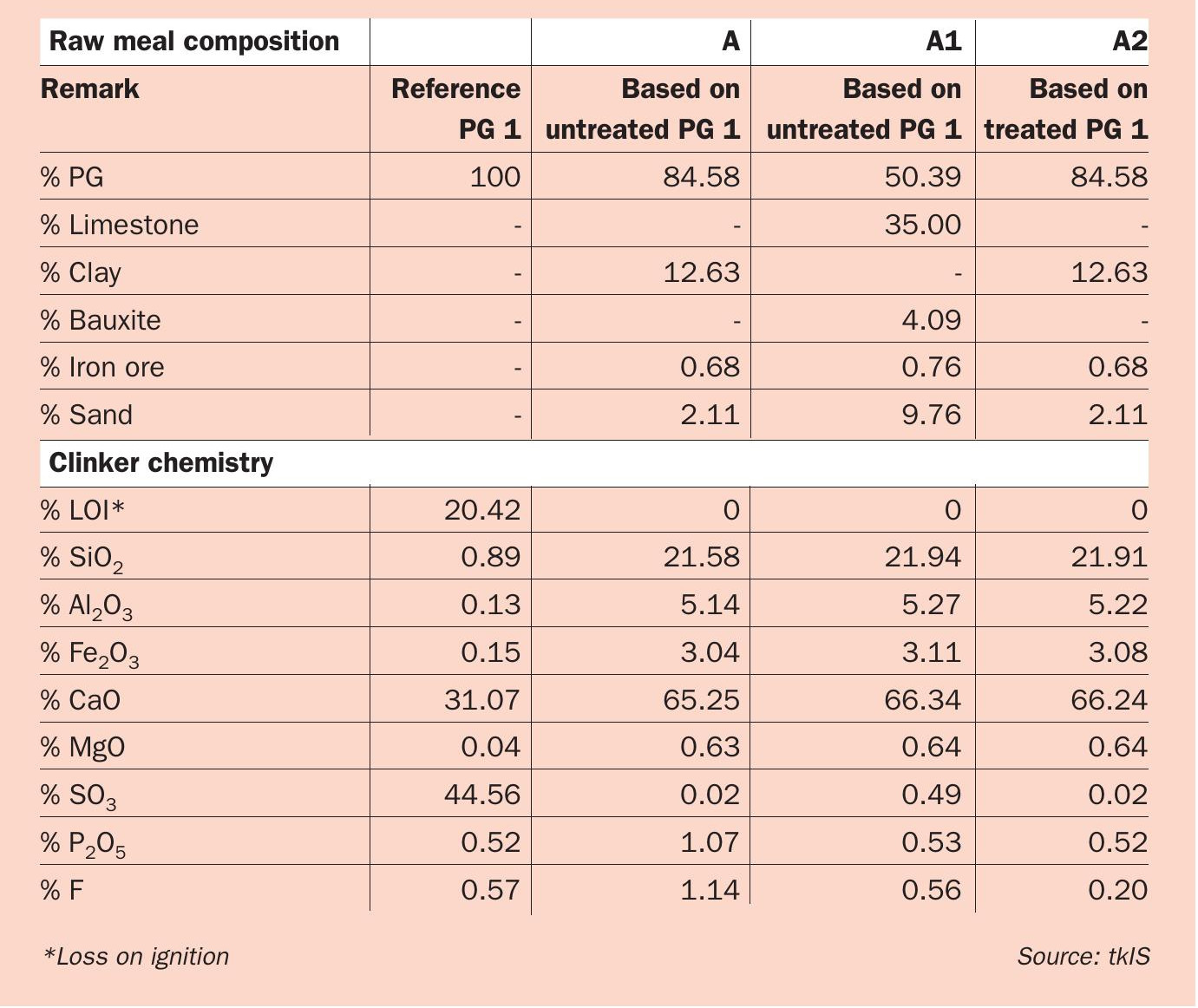
The OSW-Krupp plant in Phalaborwa, South Africa, is a typical example (Figure 1). This conversion plant, which had a design capacity of 105,000 t/a each for sulphuric acid and cement, was commissioned in 1972 and subsequently operated for 15 years.
The OSW-Krupp plants were eventually closed for economic reasons – with access to lower cost sulphuric acid being a deciding factor – although meeting cement quality and environmental standards were also both issues.
Targeting potential phosphogypsum use
Because of the large amounts of PG produced and available worldwide, research at tkIS has focused on large-scale end-markets that are capable of consuming PG in major quantities. The clinker/cement and building materials industries were identified as the two most promising target markets for PG (Figure 2).
Due to its scale, there should be sufficient demand in the international cement market for additional supply of clinker/ cement products derived from PG. Furthermore, PG also has potential as an alternative, carbon-neutral source of calcium to replace the limestone currently used in cement manufacture.
The gypsum supplied to the building materials industry is mainly sourced from natural gypsum and gypsum from downstream flue gas desulphurisation (FGD) at coal power plants. Production of FGD gypsum is, however, set to decline in future as a result of the global phase-out of coal power. This opens up a market opportunity for PG due to its potential as a raw material for plaster, stucco and plasterboard.
The cement industry and some plasterboard manufacturers have already identified PG as valuable raw material source – although, despite this, its use in these industries is still limited. However, the combination of carbon reduction goals, the emergence of the circular economy, and technology developments, have once again brought the economic potential of phosphogypsum back into focus.
PG quality – knowing what we’re dealing with
PG is generated as a by-product of highquality phosphoric acid production. Yet each individual production plant generates PG with distinctly different quality characteristics. This is largely due to the different types of phosphoric acid manufacturing process used, and the natural compositional variability of phosphate rock feed. Table 1, for example, shows the compositional differences (major and minor elements) in PG generated by four different phosphoric acid plants.
Clinker quality requirements
The suitability and potential utilisation of PG depends on a number of quality considerations. Very low values for P2 O5 and fluorine are required by both the clinker and cement industry, for example, as their presence is known to lower the development of strength in cement. Consequently, the final specification for clinker demands P2 O5 and fluorine contents of less than 0.5 percent and 0.2 percent, respectively.
The levels of silica, aluminium and certain other elements in clinker also regulate its behaviour and are therefore important to quality. It is typically necessary to add-in several materials (e.g. clay, bauxite, iron ore, sand) to the main PG feed to obtain clinker with the desired composition. This feed mixture for a clinker plant (PG plus additives) is called the ‘raw meal’.
Examples of the difference in clinker chemistry resulting from four different raw meal compositions are shown in Table 2. Trace elements (Ti, Mn, alkalis, Cl) have been omitted for simplicity.
The clinker chemistry obtained for a raw meal containing untreated PG is shown in column A of Table 2. This raw meal composition contains sufficient additives (clay, iron ore and sand) to meet the desired silica, aluminium and iron levels in clinker. However, it is clear that using untreated PG (up to 85%) does not meet the required P2 O5 and fluorine criteria.
One way to achieve the required P2 O5 content in the clinker would be to replace around 35 percent of the untreated PG with limestone (see column A1, table 2). However, the fluorine content (0.56%) would still remain too high. In any case, replacing untreated PG by limestone in this way would be uneconomical and undesirable for PG producers. Instead, the best way to achieve the target quality in the clinker (see column A2, table 2) would be to selectively treat the PG to reach a P2 O5 content of 0.24 percent and a fluorine content of 0.1 percent. This clearly shows how reducing the P2 O5 and fluorine contents of PG upstream of a clinker plant is both an achievable and viable option.
Building material requirements
The usage of PG in the building materials industry, particularly in Europe, is limited by the strict quality criteria for recycled gypsum in this market1 . The fluorine limit for gypsum used in plaster is set below 0.02 percent, for example. Because of the exacting standards, only a few PG producers worldwide are able to meet construction industry requirements without additional treatment.
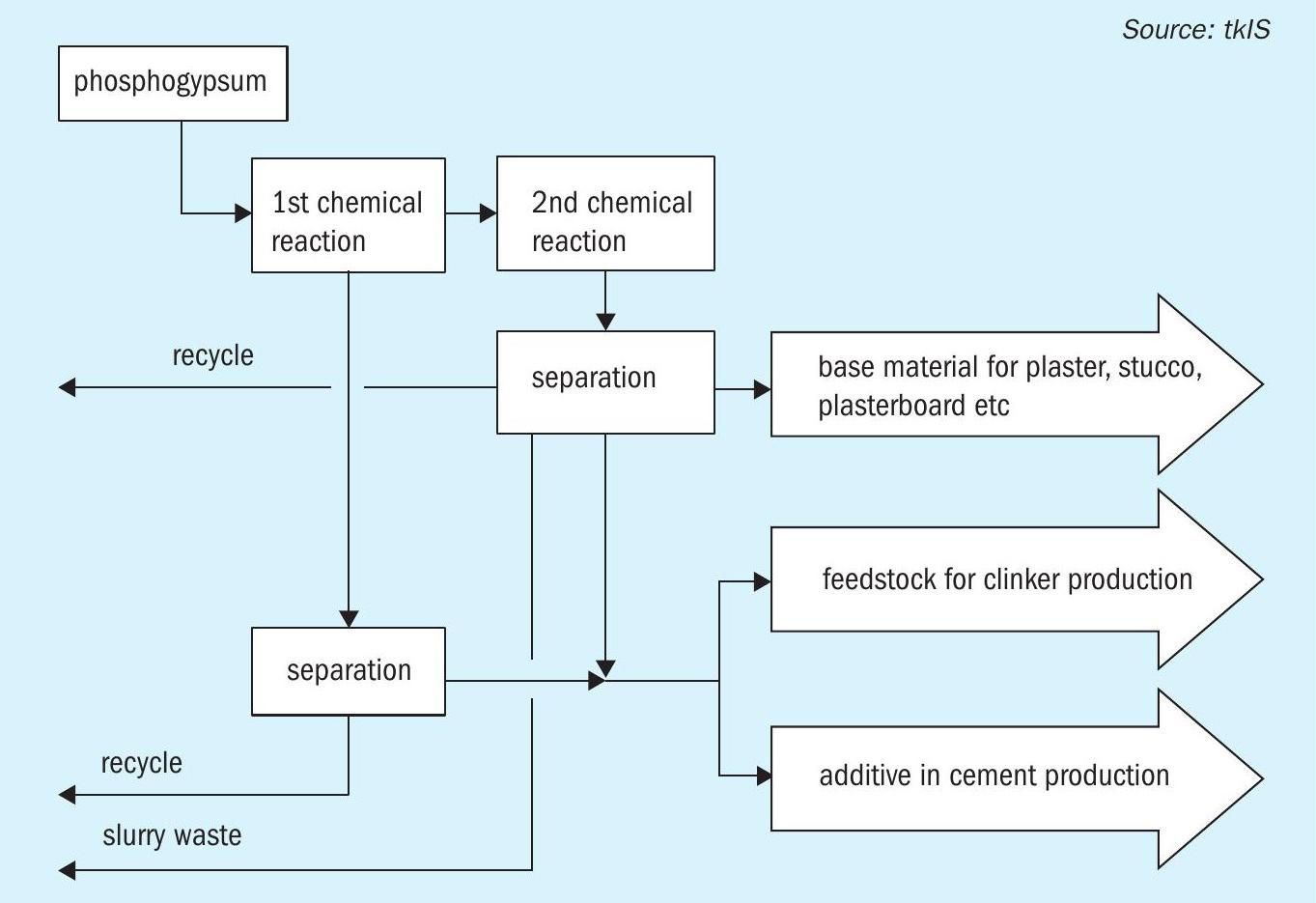
PG also contains levels of the naturally-occurring radionuclide decay products of uranium-238 and thorium-232 which were originally present in phosphate rock (Fertilizer International 478, p58). Approximately 90 percent of the original radium content of phosphate rock remains in the PG after digestion with sulphuric acid. This considerably limits its use as a building material.
Most countries use an activity concentration index (ACI) to assess building material suitability based on radioactivity. This is defined as follows according to RP 112 of the European Commission2 :

In principle, it is possible to use a bulk material with an ACI <_ 0.5 for direct use without restrictions, being equivalent to an annual radioactive dose criterion of 0.3 mSv. Generally, the ACI for building materials must not exceed I = 1, which corresponds to an annual dose of 1 mSv. For gypsum plasterboard with restricted use, the ACI must be below either 2 or 6 to meet dose criterion of 0.3 or 1 mSv a-1 , respectively.
Typical activity concentrations for PG (390 Bq kg-1 for Ra-226, 20 Bq kg-1 for Th-232 and 60 Bq kg-1 for K-40), as given in RP 112, would lead to an ACI of approximately 1.4. The usage of PG as a clinker raw material would therefore undoubtedly increase its ACI. However, the fact that clinker only makes up a proportion of cement, and an even smaller fraction in concrete, should limit the overall rise in ACI.
The above discussion demonstrates that the activity concentration index does need to be examined in detail when assessing PG for individual building material applications.
tkIS phosphogypsum treatment process
The latest PG treatment process from tkIS is based on the original conversion process for the OSW-Krupp plants. An investigation of the performance of these plants revealed that pre-treatment is required before PG is suitable for downstream production of highquality clinker/cement or other building materials. The literature on other approaches to PG treatment was also reviewed3,4 .
PG from various phosphoric acid plants was chemically and mineralogically analysed prior to developing the treatment process. Additionally, information was also gathered on the specifications and quality requirements for cement and other building raw materials. Once this data had been collated, a laboratory-scale PG treatment process was developed and tested using a step-by-step approach.
Once the laboratory tests were completed, results were transferred into a kinetic process model. This model was then used to predict the treatment process parameters needed to achieve the necessary PG quality. In this way, only a few laboratory tests are required to confirm a suitable process design for the new PG treatment method.
Treatment process results
The new PG treatment process consists of two main steps in sequence. Each step involves a chemical reaction followed by liquid-solid separation (Figure 3).
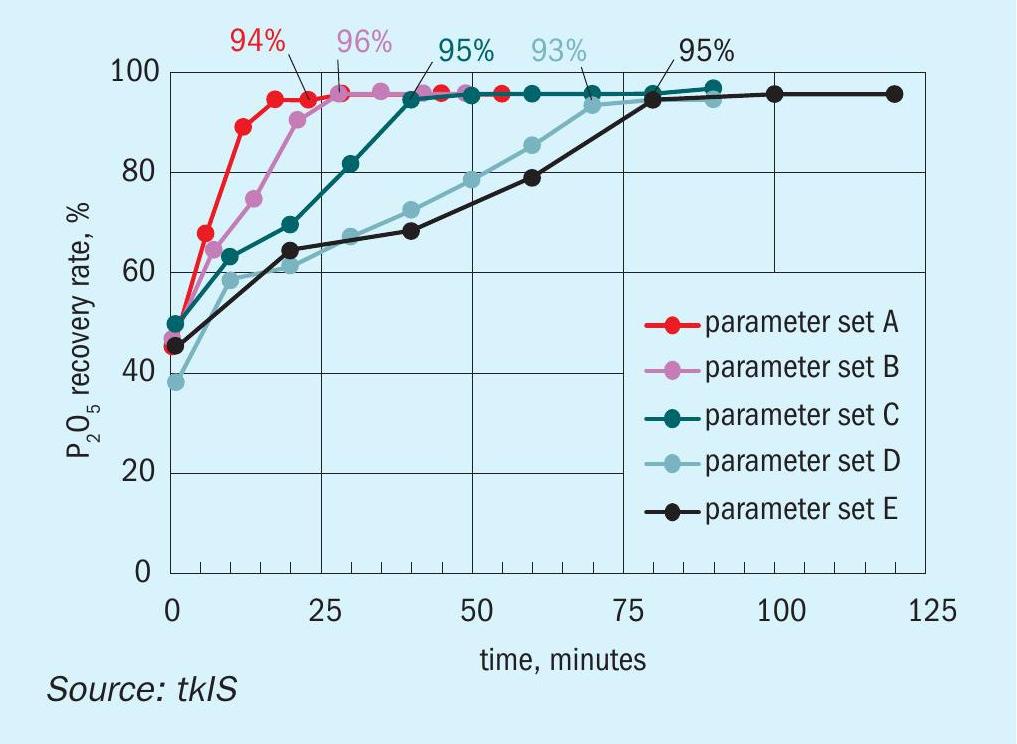
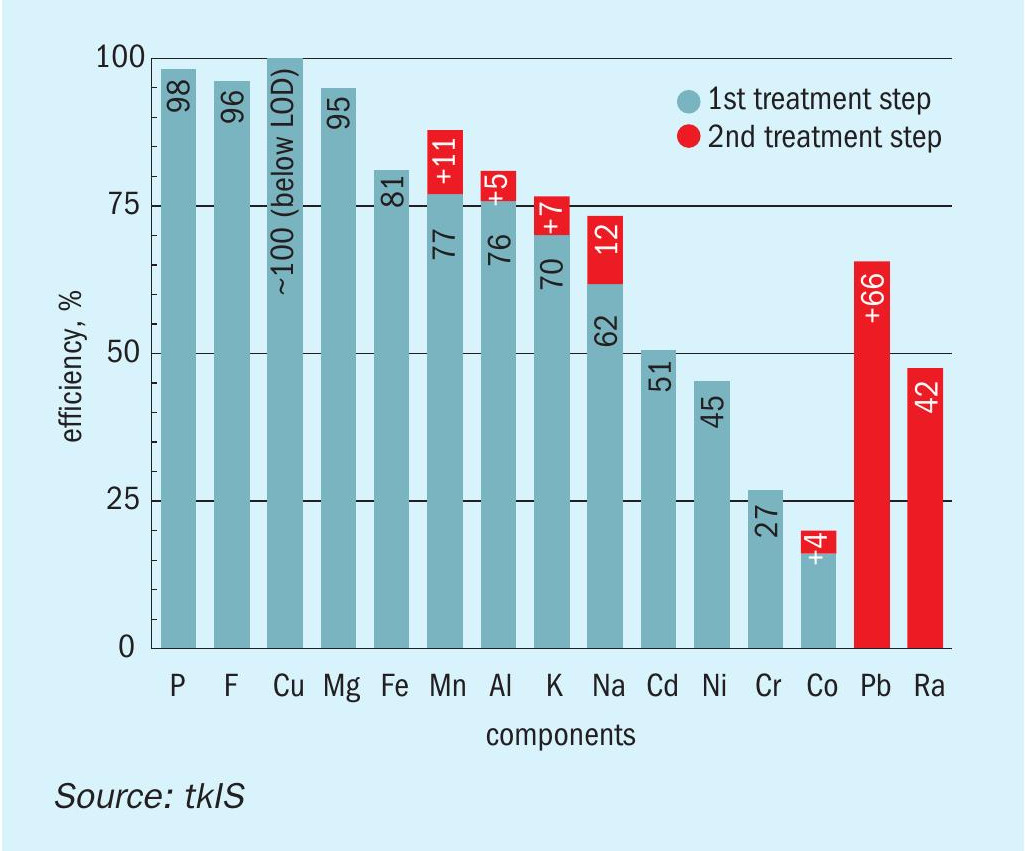
In the first treatment step, chemical impurities (P2 O5 , fluorides and others) are separated from the PG and removed in the liquid phase. This can, if required, be recycled to the phosphoric acid plant. After this step, the quality of the treated PG obtained should meet clinker/cement production requirements.
The aim of the second treatment step is to reduce radioactive components, especially radium, so the quality of the resulting PG meets building materials requirements. This treatment step is optional, depending on the composition and quality of the untreated PG.
The first treatment step performs well, being very effective at P2 O5 reduction (see four examples shown Figure 4). A typical recovery rate of significantly more than 90% of P2 O5 is possible, the exact reduction depending on the process regime. Importantly, the liquid phase containing the dissolved P2 O5 is fully reusable in the phosphoric acid plant – and therefore contributes to the overall P2 O5 efficiency of the production complex. The first treatment step has also been shown to be equally effective at reducing the fluoride content of PG.
Generally, understanding the reaction kinetics of P2 O5 recovery is critical, as this holds the key to optimising the whole process (Figure 4). Reaction conditions have a large impact on the recovery rate, with harsh reaction conditions, for example, typically leading to shorter release periods compared to mild conditions.
The overall reduction in PG impurities achieved by both treatment steps is shown in Figure 5 (first treatment step in blue, second treatment step in red).
In the first treatment step (Figure 5, blue colour), P2 O5 and fluoride are removed at efficiencies of up to 98 percent and 96 percent, respectively. In parallel with this, significant reductions in other elements (Cu, Mg, Fe, Mn etc.) are also achieved. This is highly beneficial for the potential use of PG as a plaster industry raw material. The efficacy of this first treatment step has also been tested and confirmed on PG generated by both sedimentary and igneous phosphate rock types.
The main aim of the second treatment step is radium removal (Figure 5, red colour). Treatment was found to reduce radium activity by approximately 40 percent, down from 570 Bq/kg originally to an eventual value of 330 Bq /kg. Typically, this would be expected to reduce the ACI of PG from 1.4 to 0.9 approximately. Further studies are nevertheless underway to improve and optimise the removal of radioactive elements, particularly for more radioactive types of PG.
With regard to radioactivity, and despite what is achievable technically, decisions on the ultimate usage of treated PG will still require discussions between manufacturers, users and authorities.
Summary and outlook
thyssenkrupp Industrial Solutions (tkIS) has conducted intensive research with the aim of developing a technology to convert phosphogypsum (PG) into a valuable product for the circular economy. A two-step treatment process for the controlled reduction of impurities in PG has been devised and tested at laboratory-scale. This has shown that treatment can successfully achieve reduction rates of more than 95 percent for both P2 O5 and fluoride. Promisingly, the radium activity of PG was also reduced by more than 40 percent in initial tests – with higher reduction rates expected in future.
Building on these positive lab-scale results, the next step of process development will scale-up capacity and optimise energy consumption. A strategy for achieving full integration of the PG treatment process within existing production plants will also be pursued. The opportunities for plant integration will be evaluated in cooperation and partnership with interested phosphate producers.
References