Nitrogen+Syngas 372 Jul-Aug 2021
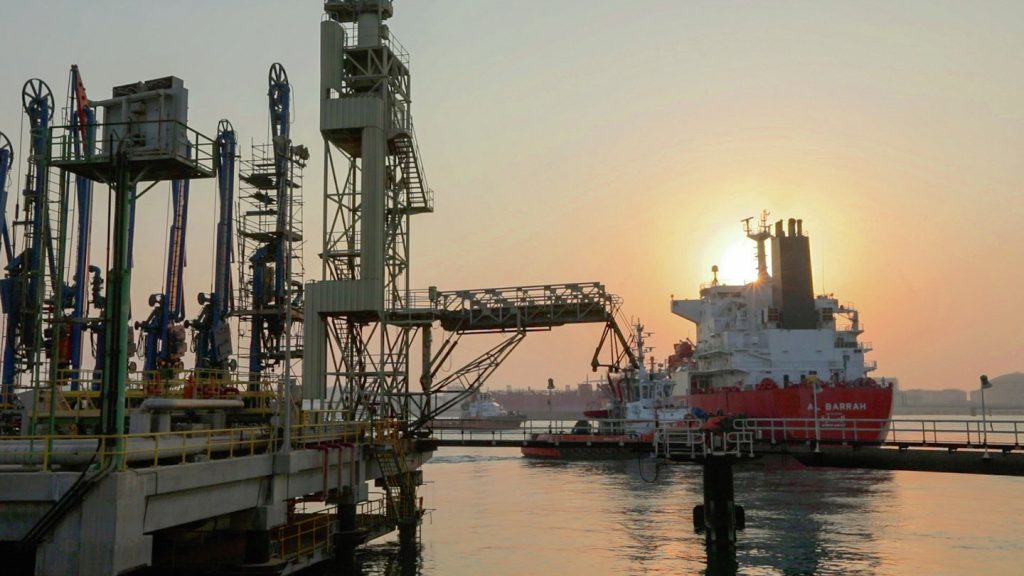
31 July 2021
Ammonia to burn
AMMONIA FUEL
Ammonia to burn
Plans to decarbonise power production and shipping are leading to increasing interest in using ammonia as a fuel, but technical and economic barriers still remain to be overcome.
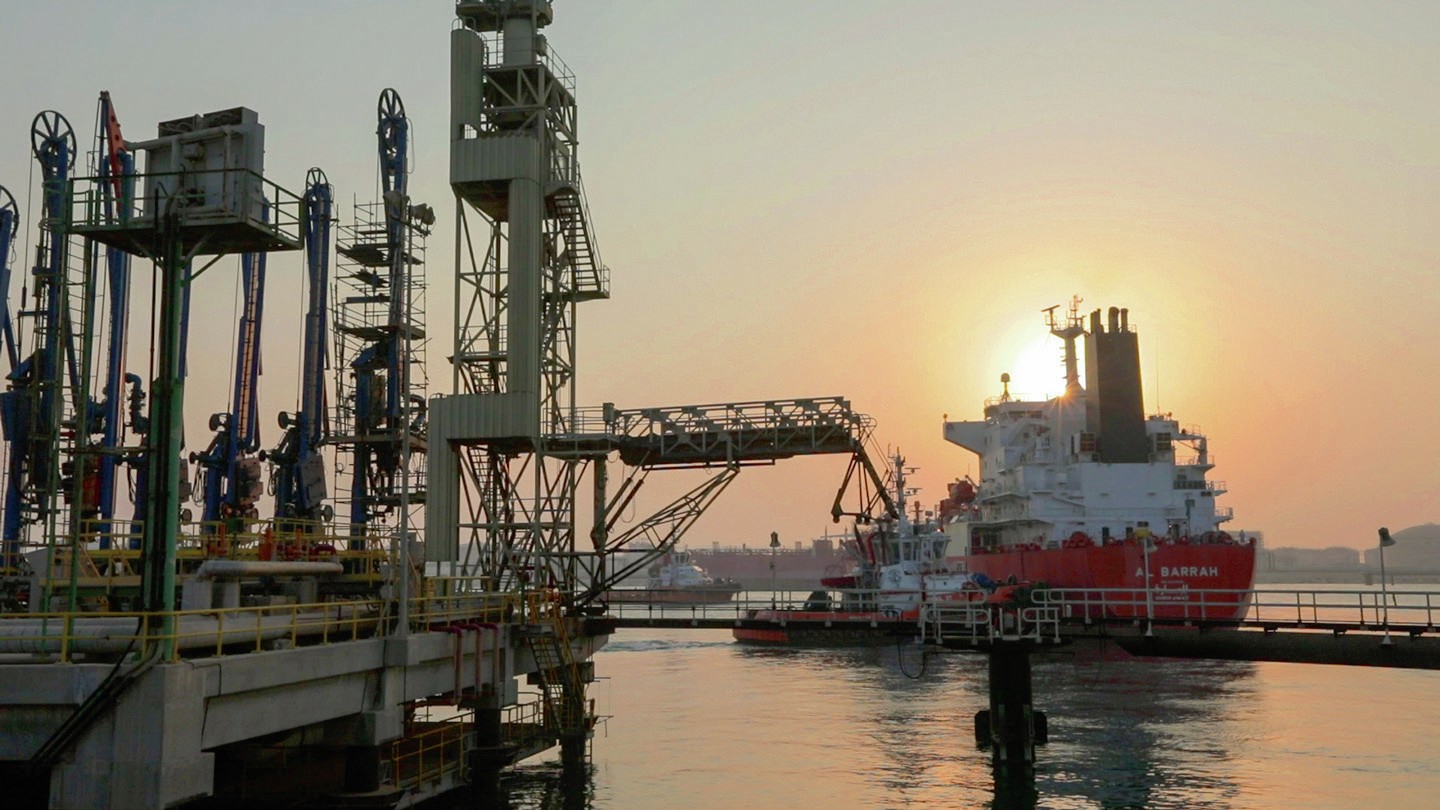
Ammonia burns. In some ways it is as simple as that. Not as energetically as hydrocarbons – its energy density (higher heat value) is about 22.5 MJ/kg; about half that of a typical hydrocarbon like gasoline or diesel – but nevertheless about the same as methanol and significantly more than hydrogen, and certainly close enough to existing fuels that it could be used to run a vehicle. Ammonia-powered engines have a long history, and in the 1930s and 40s there were some experimental ammonia-powered cars. Ammonia Casale actually built an engine designed to run on a mixture of ammonia and coal gas in the 1940s, and which was used to run buses in Belgium during World War II when gasoline was in short supply. Gasoline currently costs around $700/tonne in the US ex-refinery, before taxes and distribution costs, while ammonia on a tanker can currently be had for $300/tonne f.o.b., before shipping and handling costs, so per unit of energy, it’s even roughly on a par.
These facts alone however are not enough to encourage the use of ammonia as a vehicle fuel, because it also has significant drawbacks. While gasoline is flammable to the point almost of explosion, ammonia in gaseous form is highly toxic if breathed in, and in order to maintain it as a liquid it must be cooled and stored below -33°C at atmospheric pressure or compressed to around 10 bar (raising the boiling point to about 25°C), or some combination of the two, increasing both the dangers and costs of its transport and storage. Furthermore, while the basic equation for combustion of ammonia looks quite clean:
4NH3 + 3O2 → 2N2 + 6H2 O
producing in theory only inert nitrogen and water, in practice nitrogen oxides (NOx) are also produced in significant quantities, equally toxic if breathed in, as well as being, in the case of N2O, a gas with a very high global warming potential. These must be scrubbed from any ammonia engine exhaust, although selective catalytic reduction using ammonia generated from a solution of urea is now a standard part of most large goods vehicles in North America and Europe, and increasingly of diesel passenger cars, and adds only marginal additional cost.
But what has brought about ammonia’s current vogue as a potential fuel source is the potential for it to be made with low carbon emissions, either from hydrogen generated from water electrolysis using renewable energy, or from conventional coal or methane feedstock with carbon capture and storage – ‘green’ and ‘blue’ ammonia respectively. While at present very little ammonia is actually made this way, the number of new low carbon ammonia projects continues to multiply almost daily, with some like Yara’s projected conversion of its Porsgrunn plant, and large scale projects in the Middle East such as those in this issue’s Industry News section in the UAE, Oman and Saudi Arabia, as well as interest in Australia, there could by several million t/a of renewable ammonia being produced by 2030. Industries like shipping, as well as the Japanese power sector, which are looking towards a zero carbon future but puzzling over how to get there, have begun to fix on low carbon ammonia as the solution to their problems.
Power generation
If ammonia can be burned, then presumably it can be used in a gas turbine to drive electricity production. However, there had been little practical work done on this until a few years ago. Then in 2014, Hideaki Kobayashi, professor at the Institute of Fluid Science at Tohoku University in Sendai, Japan, in conjunction with the country’s National Institute of Advanced Industrial Science and Technology (AIST), built the first working direct ammonia-powered gas turbine which generated electricity. As ammonia’s range of combustion in air is fairly limited (between 15-25%) he had to develop a spiral mixing system to ensure a stable concentration of ammonia in air, and the turbine uses extra turbine blades that generate a high-pressure, high-temperature fuel mixture.
Japan is interested in ammonia as a potential energy carrier, and plans to introduce ammonia into the fuel mix for thermal power generation, as part of its measures to cut CO2 emissions and reach carbon neutrality by 2050. As part of its Green Growth Strategy, the government is targeting ammonia imports either for conversion back to hydrogen and nitrogen (using ammonia as a hydrogen carrier) or by burning ammonia directly in power production.
The government push is leading to major players committing large sums on research and development. Dr Kobayashi’s gas turbine generated 40 kW of electricity, but in March 2021 Mitsubishi Power, part of Mitsubishi Heavy Industries (MHI), announced that it had begun development work on a 40 MW 100% ammonia powered gas turbine system. The company says that it is targeting commercialisation in or around 2025. Among the challenges it admits it needs to overcome is dealing with the generation of nitrogen oxides, and Mitsubishi Power says that it is aiming to resolve this by combining selective catalytic reduction with a newly developed combustor that reduces NOx emissions, for installation in the Company’s H-25 Series gas turbines.
Meanwhile, in May 2021, Yara International signed a memorandum of understanding with Japan’s JERA Co., the country’s largest power generation company, to collaborate on the production, delivery and supply chain development of blue and green ammonia, to enable zero-emission thermal power generation in Japan. Yara is aiming to sequester CO2 at its ammonia plant in the Pilbara region of Western Australia, enabling the production and supply of blue ammonia to JERA, as well as potentially jointly developing new green and blue ammonia capacity and optimising the logistics of shipping ammonia to Japan. JERA produces about 30% of Japan’s electricity.
Japan’s Green Ammonia Consortium, which includes Kansai Electric Power, Shell Japan, trading houses such as Mitsui and Marubeni, as well as Mitsubishi Heavy Industries, says that it expects 1% of Japan’s electricity to come from blue and green ammonia combustion by 2030, potentially rising to 10% by 2050. Initially, this will involve burning ammonia alongside coal to generate electricity. An experiment at Chugoku Electric Power in 2017 successfully burnt ammonia and coal in an existing power station, and JERA says that it will convert its 1 GW Hekinan coal-fired power station in Aichi, central Japan, to a 20% ammonia feed during 2024-25. By 2030, Japan expects to be importing an extra 3 million t/a of ammonia for power generation. Developments outside Japan are still limited at present, however, and the country is becoming something of a guinea pig for the development of renewable ammonia as a power plant feed.
Marine fuel
The International Maritime Organisation (IMO) has set a goal of reducing the total greenhouse gas (GHG) emissions from international shipping by at least 50% by 2050, compared to 2008 levels. In addition, a target has been set to reduce the carbon intensity of shipping by 40% by 2030, thus emphasising the need for the rapid introduction of existing and new low carbon technologies. This in turn seems to have galvanised some segments of the maritime industry to start looking seriously at renewable ammonia as a fuel candidate. Among the prime movers in this has been Finnish marine engine manufacturer Wärtsilä, which, in conjunction with the European Commission and European research initiative the Waterborne Technology Platform, has been working on four-stroke ammonia internal combustion engine designs, hoping to reach the stage of field tests as soon as 2022. The company is also developing ammonia storage and supply systems to install ammonia fuel cells on Eidesvik Offshore’s supply vessel Viking Energy by 2023, part of the EU project ShipFC. After its conversion, Viking Energy is expected to become the first carbon-free ammonia-powered vessel in the world. Equinor will use it for supply operations on the Norwegian continental shelf to help cut its supply-chain emissions. Yara will supply the ammonia using renewable power as part of the ShipFC consortium.
Meanwhile, MAN Energy Solutions is expecting to have a two-stroke ammonia engine ready to deliver by early 2024, Kirkeby said. By the following year, the company aims to offer retrofit conversions to allow existing two-stroke engines to use ammonia.
As with power plant developments, Japan has also been working on ammonia as a shipping fuel. Japanese trading house Itochu has signed a memorandum of understanding with Dutch oil storage and terminal operator Vopak for a feasibility study concerning the development of ammonia supply infrastructure for use as a marine fuel for vessels in Singapore. Itochu already operates an ammonia storage and handling facility at Singapore’s Banyan terminal, and is now looking at the possibility of building offshore facilities such as a floating storage tank and an ammonia fuel supply vessel. Itochu is also already involved in a project in Japan to develop ammonia supply infrastructure and launch ammonia-fuelled commercial vessels. Last year, Japanese shipping company NYK Line, shipbuilder Japan Marine United Corporation (JMU), and ClassNK signed a joint research and development agreement for the commercialisation of an ammoniafueled ammonia gas carrier that would use ammonia as the main fuel, in addition to an ammonia floating storage and regasification barge.
Finally, shipping leviathan Maersk said in a 2019 report that it sees ammonia, along with biogas and alcohol, as one of its three main “commercially viable” candidate fuels for low carbon shipping.
The potential for ammonia in the marine industry is great; the International Energy Agency (IEA) has suggested that its use for shipping could reach 130 million t/a tonnes by 2070 – on the same scale as its use for fertilizer. Figure 1 shows estimates from the American Bureau of Shipping, which suggests that one third of all shipping fuel consumption could be represented by ammonia by 2050, which would again be well over 100 million t/a at current fuel consumption rates. At the moment, however, its use is limited to a few research and development vessels, and its eventual uptake, as with the power sector, is very much dependent on the availability of blue and green ammonia – while ‘grey’ ammonia is cheaper than most alternative marine fuels, blue and green ammonia are not, and the use of ammonia does not justify the expense of a switchover from a conventional fuel unless it is a zero carbon fuel option.
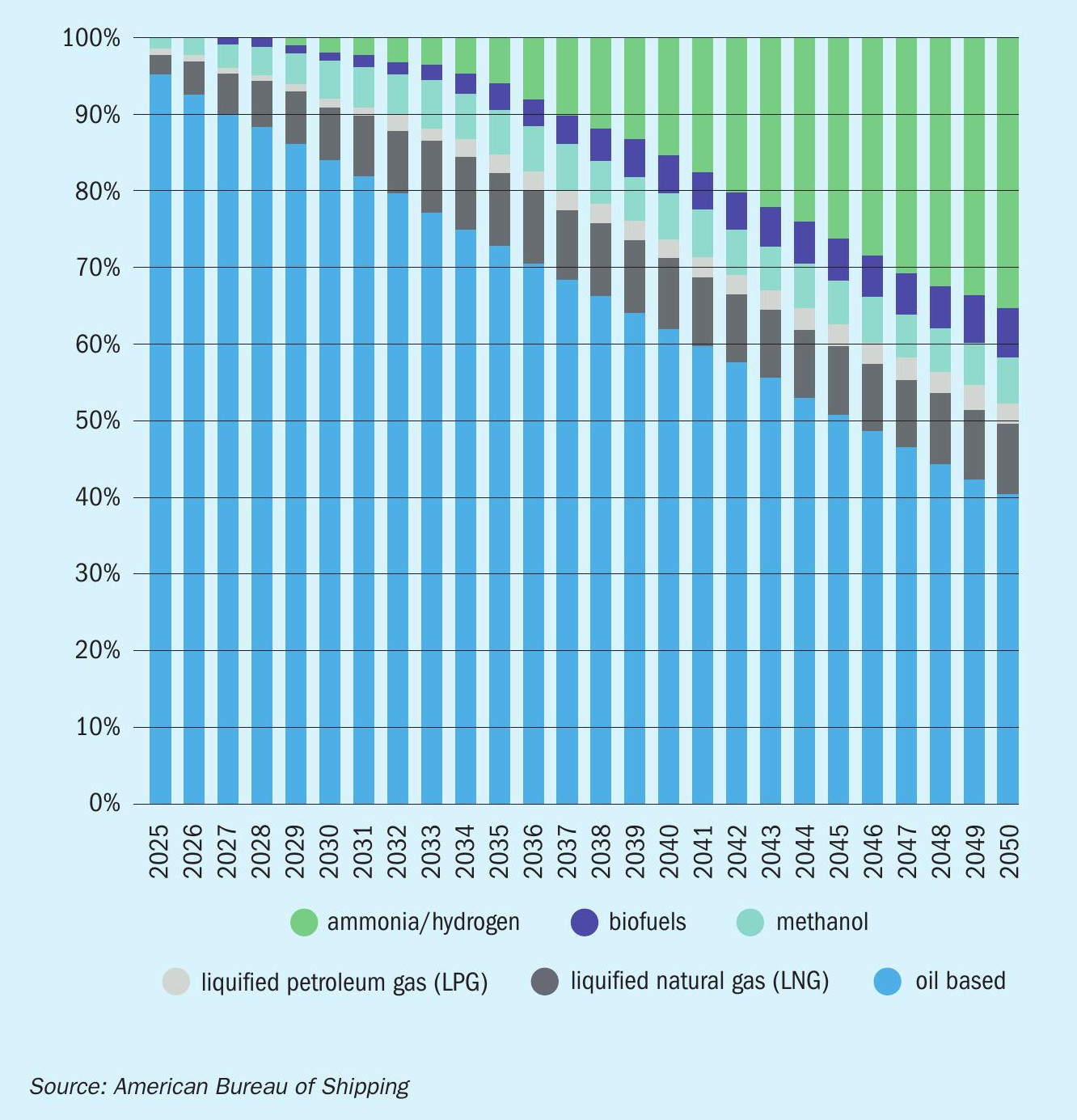
Barriers to commercialisation
The main barriers to commercialisation of green/blue ammonia as a low carbon fuel are threefold; technical, economic and ensuring sufficient supply. Of these three, the technical seems to be the least daunting; there is a considerable amount of research and development under way by some major players in their respective industries – companies like MAN and Wärtsilä on the shipping side, and Mitsubishi and JERA on the power plant front. The question is merely one of engineering, and there do not seem to be any insuperable barriers on the horizon. Removal of nitrogen oxides from an exhaust stream is already a well understood and proven technology.
The economic side is potentially more challenging. At present, fully ‘green’ ammonia costs two to four times as much to make as conventional ammonia. CF Industries is currently planning to convert part of the feed for an ammonia train at its Donaldsonville plant in Louisiana by 2024, by which time it will be producing 20,000 t/a of green ammonia using hydrogen from electrolysis with electricity provided from renewable sources. CF puts the cost of producing green ammonia at $500/t, significantly more than the present f.o.b. cost. The company suggests that it could reap a reward in terms of premium prices for green ammonia. But this in turn pushes the cost onto downstream consumers and could raise the price of, e.g. shipping, were it to become a shipping fuel. Still, electrolyser costs have already come down tenfold over the past decade, and as renewable power continues to be introduced at scale, so unit costs are likely to come down still further. BP suggests that by 2050, the cost of renewable energy will have fallen by 30-70% compared to its current value – already cheaper than conventional fuels in many markets.
In the meantime, blue ammonia might be a cheaper bridging option in the short term, and CF Industries, along with Nutrien in North America, is also looking at large scale conversion to CCS for its ammonia production. Plants in the US Gulf Coast are near to established oil and gas fields and so could use CO2 for enhanced oil recovery, while taking advantage of tax credits for CCS. Since 2013, Nutrien has been selling about 250,000 t/a of CO2 into the EOR market from its Geismar fertilizer plant in Lousiana. Saudi Arabia, Russia, and several other countries are either already producing or actively converting capacity to CCS, mainly for enhanced oil recovery. Saudi Aramco recently shipped 40 tonnes of blue ammonia as part of a demonstration shipment to Japan, with 30 tonnes of CO2 captured during the process designated for use in methanol production at SABIC’s Ibn-Sina facility and another 20 tonnes of captured CO2 being used for enhanced oil recovery at Aramco’s Uthmaniyah field. While piping to handle acidic CO2 and pump it back into oil reservoirs is an expense, it is only a marginal increase in cost to the cost of producing conventional ammonia. It seems likely that we will see many more such projects over the next few years, as a market develops for low carbon ammonia.
The third barrier, however, may be the most significant – that of producing sufficient low carbon ammonia to meet anticipated demand. Japan is looking at 3 million t/a of ammonia demand for power generation by 2030, and JERA is working with Yara to source ammonia for Japan’s power industry. Taken with other blue and green ammonia developments, such as Yara’s Porsgrunn conversion, and the recently announced projects in the UAE to add 1.2 million t/a of blue and green ammonia before the end of the century, this looks achievable. But if ammonia did take off as a shipping fuel in the way that Figure 1 suggests, the prospect of 100 million t/a of demand by 2050 would require a wholesale change in the industry, and that in turn might require the economics, and possibly even the demand, to come first. And, just as ammonia production must currently compete with the power industry for natural gas and coal feedstocks, so in future it might have to compete with the power industry for renewable electricity. Still, if the shipping industry feels it has no alternative (developments in electrically powered vessels are even more in the experimental phase than ammonia) in order to achieve the IMO low carbon goal, that might just be enough of a push to develop blue and green ammonia plants at the scale required to achieve it.