Sulphur 403 Nov-Dec 2022
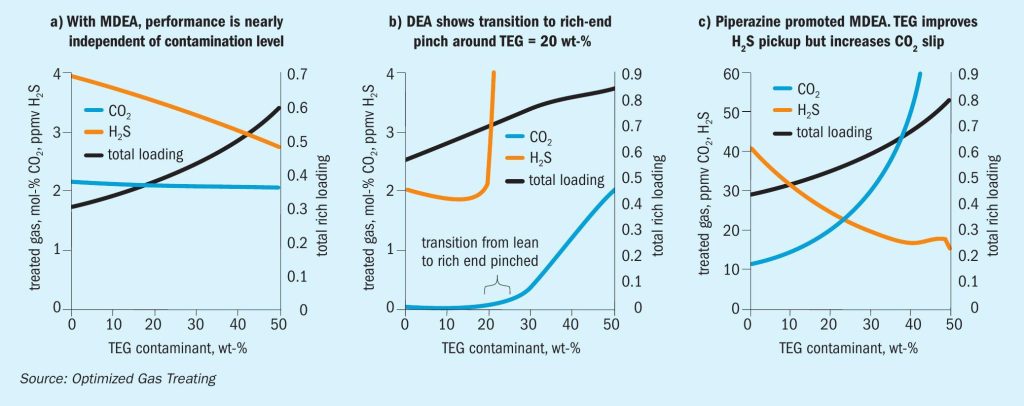
30 November 2022
Effect of amine contamination by TEG
GAS TREATING
Effect of amine contamination by TEG
The capabilities of OGT’s ProTreat ® simulator were recently enhanced by the addition of gas treating with hybrid solvents. The functionality was developed generically to make it as broadly applicable as possible. In this article, Ralph H. Weiland of Optimized Gas Treating investigates the effect of TEG as a contaminant on the performance of DEA, MDEA, and piperazine-promoted MDEA in a gas treating application.
Hybrid solvents are a class of extractive agents in which, typically, some of the water and amine content of a conventional amine-based solvent is replaced by a physical, non-aqueous, non-reactive component. The earliest commercial example is the solvent Sulfinol-D ™ as developed and licensed by Shell Catalysts & Technologies over the last 60 years.There, some of the water and some of the amine (diisopropanolamine, DIPA) are replaced by the purely physical solvent, sulfolane. Later, Sulfinol-M ™ , and Sulfinol-X ® were developed for other gas treatment applications. But, by no means is the inert component in an amine-containing chimeric solvent limited to sulfolane. In principle, any inert solvent could be a candidate. In what follows, triethylene glycol (TEG) replaces sulfolane in the solvent, with a view to quantifying the effect of TEG as a contaminant on the performance of DEA, MDEA, and piperazine-promoted MDEA (referred to as pMDEA here) in a gas treating application.
Glycols are used for gas dehydration, so glycol-contaminated solvents are fairly rare in the refining industry because drying gases is not a usual concern there. However, finished natural gas usually enters a pipeline system where there are strict restrictions on the permitted moisture content of the entering gas. Dehydration is done following amine-based acid gas removal, so carryover is not usually responsible for contamination by glycols, but there are numerous other routes, including misaligned piping, deliveries of the wrong material placed into the wrong tanks, and tank trucks not cleaned out after the previous delivery. The purpose of this article is to shine light onto the question as to how serious the effects of contamination really are on the performance of an amine unit.
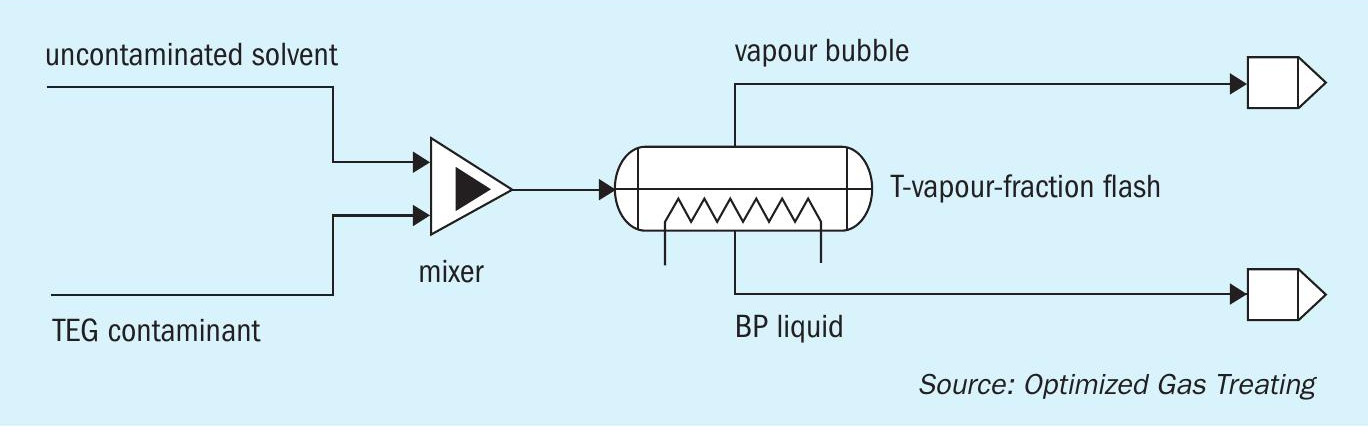
The approach uses the OGT | ProTreat ® process simulator. ProTreat’s capabilities were recently enhanced by the addition of gas treating with hybrid solvents. The functionality was developed generically to make it as broadly applicable as possible. It is not limited to sulfolane but includes any non-reactive solvent component in the software’s database, e.g., dimethyl ether of various polyethylene glycols, sulfolane, MEG, DEG, and TEG.
Important parameters
There is a long list of parameters important to absorber and regenerator performance in gas processing, but only a handful stand out as potentially more sensitive to significant concentrations of contaminant. These include acid-gas vapour-liquid equilibrium (VLE), solvent capacity, and circulation rate. All are interrelated. VLE is obviously important because it:
- controls the driving force for absorption rate of the acid gases;
- sets the maximum achievable treating level in a column and for a component whose removal is directly related to solvent lean loading (moles of removed component per mole of total amine), a normal and common situation referred to as a lean-end pinched column.
Other solvent properties do not determine the maximum achievable treating level, but they play a role in determining how quickly (or in how short a column) that level can be reached. Solvent capacity is directly related to the amine dilution resulting from the addition of an inert solvent component. Contamination by TEG will always lower solvent capacity simply because the amine strength is reduced, although this can be mitigated if the hybrid has increased capacity for acid gas because of more favourable VLE. Thus, before doing simulations of an actual column, examining how contamination by TEG affects VLE is in order. The assessment was done using the ProTreat simulator and treating the amine-water-TEG mixture as a hybrid solvent. This allows proper accounting to be done for interactions between various molecules at high concentrations.
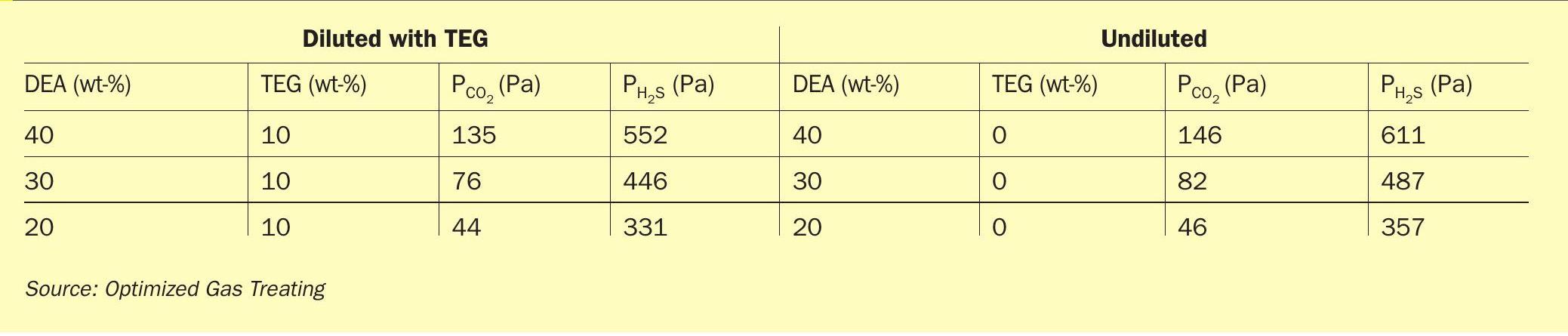
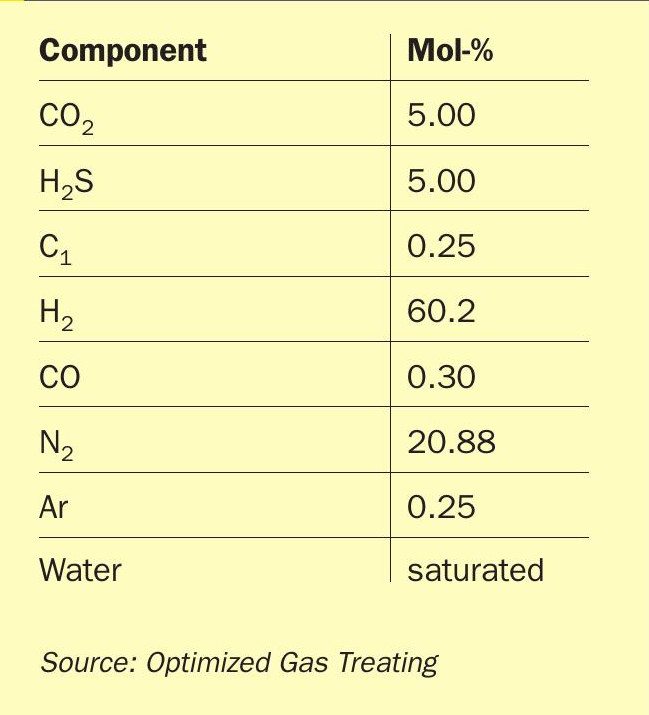
Approach to VLE calculations
Simulations were designed to mimic contamination of an original TEG-free solvent. Fig. 1 shows how a specified mass flow rate of TEG is added to a given mass flow of uncontaminated (virgin) solution to produce a contaminated mixture. With temperature specified and the vapour fraction set to a very small number (say 10 – 6 ) the liquid is forced to its bubble point without generating enough vapour to alter its composition.
Vapour-liquid equilibrium of CO 2 and H 2 S
OGT | ProTreat ® already has a solid electrolyte thermodynamics framework for aqueous amine systems. Hybrid solvents just add another inert (nonreactive, physical) liquid component to the solvent. A rigorous thermodynamic model was implemented, originally focussing on sulfolane as the inert solvent component but extended to the glycols, to represent the VLE of mixed solvent systems that can simulate any arbitrary combinations of chemical and physical solvents. Chandran et al. 1 give examples for the amine-sulfolane system. The same approach for the amine-TEGwater system accurately represented acid-gas phase equilibrium over several orders of magnitude of partial pressure, spanning a wide range of temperatures, from absorber to regenerator conditions and corresponding to a number of different solvent formulations and a range of acid gas loadings.
As more and more TEG is added to the amine solvent, the amine becomes increasingly dilute, regardless of whether the diluent is TEG or water. Table 1 shows calculated acid gas partial pressures for a typical amine (DEA) at commercial strengths (20-40 wt-%) loaded to 0.1 mol/mol with either acid gas at 45°C, and with TEG contamination of 0 (no contamination) and 10 wt-%. In the interest of space, results are not shown for mixed gases, or other amines, blends, temperatures, or loadings. Suffice it to say all results are consistent with the trends in Table 1 – decreasing amine strength results in decreased acid gas partial pressure, in the case of CO 2 by a factor of three by diluting from 40 to 20 wt-% DEA. In other words, the act of dilution at a given acid gas loading causes a given amine formulation to exert lower acid gas partial pressure, allowing it to achieve a higher purity gas.
Closer inspection between the diluted and undiluted cases in Table 1 reveals that dilution even with just 10 wt-% TEG lowers the H 2 S backpressure by nearly 10%. These observations suggest that based on phase equilibrium alone, we should expect that amine solvents contaminated with significant TEG concentrations might perform somewhat better than the virgin solvent in terms of the lowest achievable acid gas content in the treated gas. Granted though, the differences are not huge. It may be noteworthy that dilution of the 40 wt-% virgin DEA solvent with water (to 30% and 20%) is directionally similar to dilution with TEG from 0% TEG to 10 wt-% TEG.
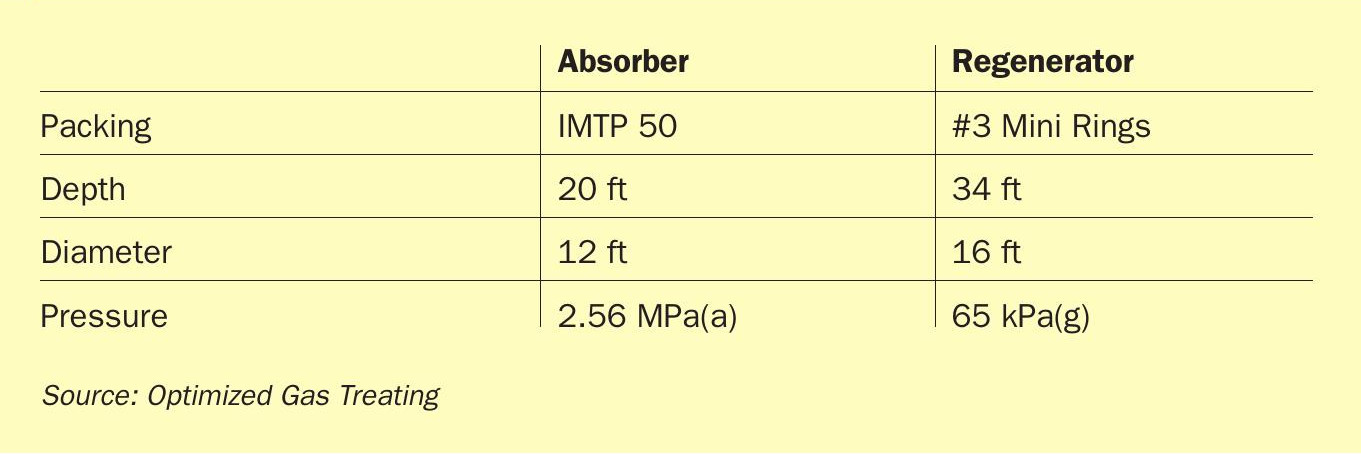
Effect of TEG contamination on CO 2 and H 2 S VLE in DEA, MDEA, pMDEA
A wealth of information can be garnered from simulation based on reliable chemistry and physics of the processes occurring, much more than can be presented in a short technical article. Thus, the presentation is limited to representative cases only. All results for the VLE calculations presented here are at 45°C for 50 wt-% MDEA, 30 wt-% DEA, and (42 wt-% MDEA + 3 wt-% piperazine) as a piperazine-promoted MDEA (pMDEA) solvent.
Figures 2-4 show the simulated effect of contamination by up to 50 wt-% TEG on CO 2 and H 2 S equilibrium partial pressures over MDEA, DEA, and pMDEA solvents. The decrease in acid gas backpressure can be up to a factor of nearly three and is similar for CO 2 and H 2 S, although the H 2 S backpressures generally decrease in the order DEA <pMDEA, MDEA at gas loadings of 0.1-0.5 mol/mol. Of course, contamination at 50 wt-% TEG could be catastrophic because roughly a doubling of solvent circulation rate might be needed to give a similar level of treating.
Mass transfer rate-based simulation
Simulating a separation process with ideal stages requires only accurate vapour-liquid equilibrium. The column internals are irrelevant, so the column could as well be empty as far as the effect of tower internals on the separation found from stagewise calculations is concerned. Physical and transport properties are needed only for tower sizing calculations. The advantage is simplicity. The disadvantage is a calculated result that is extremely hard to translate into the reality of the separation in real equipment. There is inherent inability to translate theoretical stages into physical equipment.
When the simulation is mass transfer rate-based the physical, mass transfer, and hydraulic characteristics of the tower internals are merged with reaction kinetics and phase equilibrium thermodynamics and calculations are all done simultaneously. Mass transfer rates between the phases are calculated in a way that parallels how heat exchanger calculations have been done for at least a century. The methods are rigorous and reliable, they are based on sound scientific and engineering principles, and they provide simulated performance of extraordinary accuracy and reliability with absolutely no need to make any translation from theoretical stages to real internals 2 .
Mass and heat transfer behaviour of the tower internals are characteristics of the internals themselves, but they also depend on certain properties of the phases, and of the species being transported between them. Properties include density, heat capacity, phase viscosity, diffusion coefficients of species in the gas and liquid phases. Properties depend on the species present and their concentrations and usually require mixing rules to transition from pure components to mixtures. From a mass transfer standpoint, however, if a tightly designed system becomes contaminated with glycol, the loss in solvent capacity overrides all other considerations. Mass transfer rates and solvent properties become moot – solvent capacity limitations force the absorber to become rich-end pinched.
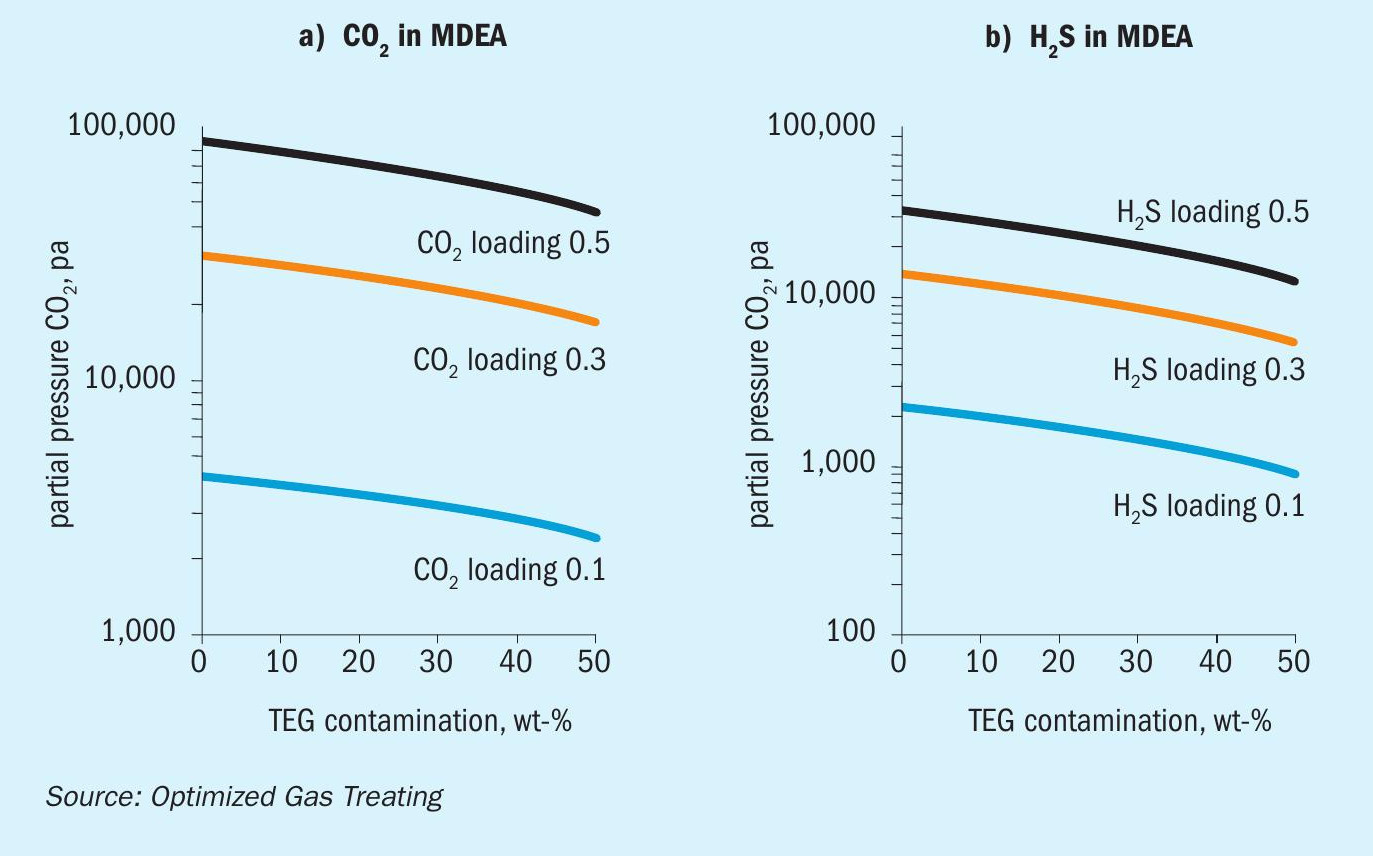
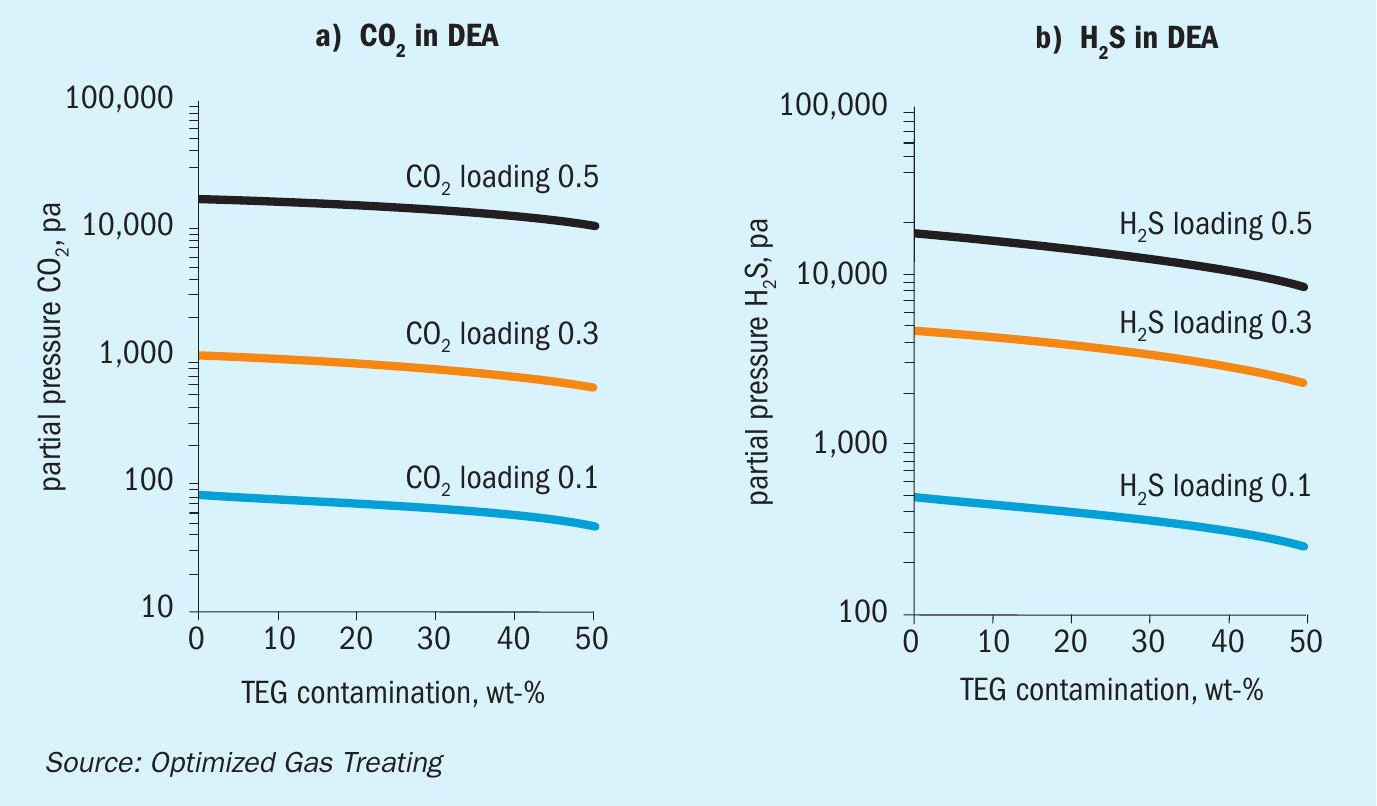
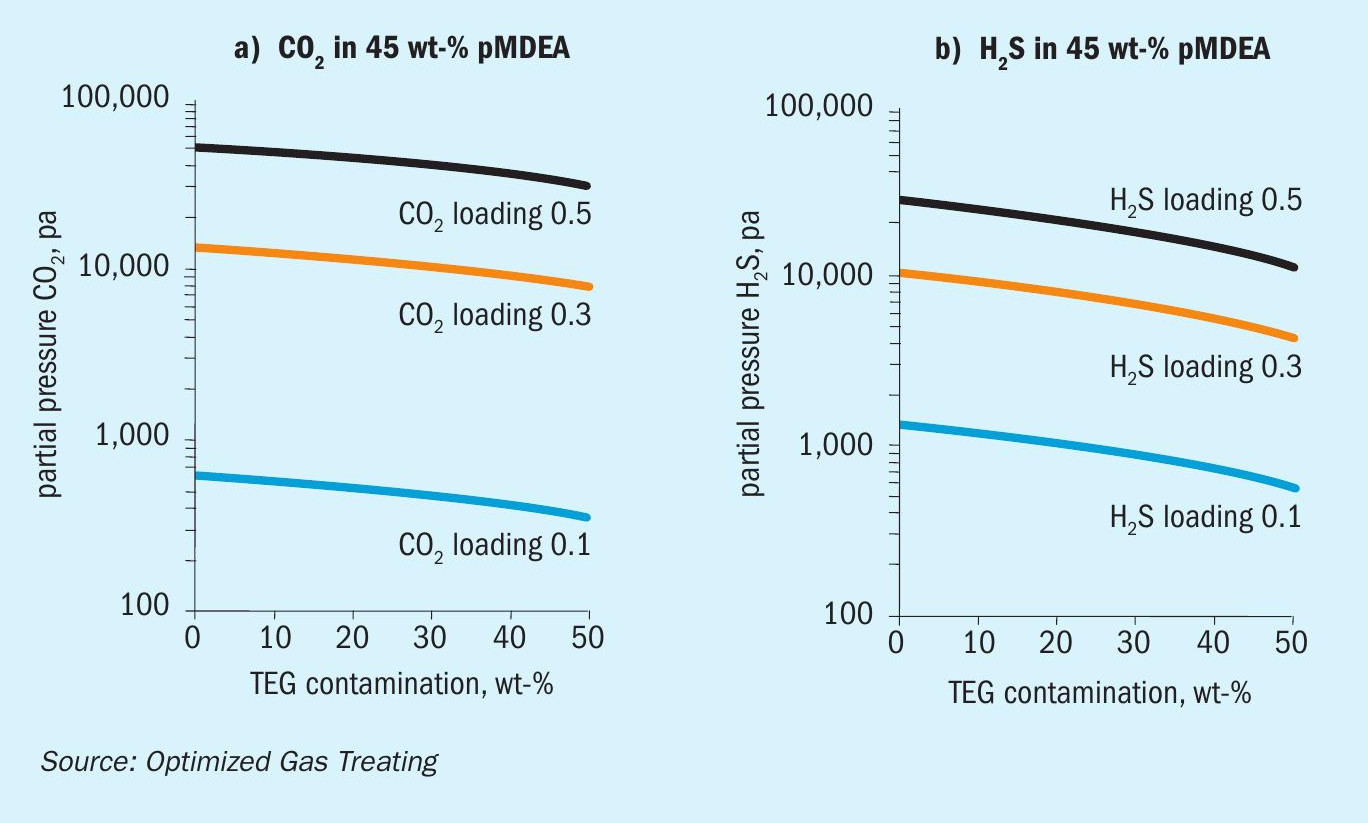
Effect of TEG contamination on treating
As a gas treating solvent, the amine content is reactive toward CO 2 and H 2 S, but water is an essential ingredient because it allows the amine to react with the acid gases in the first place (aqueous and non-aqueous amine chemistries are quite different). Water allows the amine to dissociate and form ionic reaction products, thereby providing the solvent with high capacity to hold a substantial amount of acid gas. The inert part of the solvent (TEG) can also solubilise acid gases but not to nearly the extent as the amine because it is nonreactive.
For any solvent, the number and range of parameters that could be studied are prohibitive so, to keep the discussion manageable the same raw gas (Table 2) and identical column (absorber and regenerator) details (Table 3) were used for each of the three solvents explored. Solvent rates and reboiler duties are as noted (Fig. 5).
MDEA
Models were run using the OGT | ProTreat ® simulator on 50 w-t% MDEA virgin solvent using 0, 10, 20, 30, 40, and 50 wt-% TEG contamination at a total flowrate of 175 L/s. Regenerator reboiler duty was 175 GJ/h. Flowrate and reboiler duty were selected to reject as much CO 2 as possible while having the H 2 S level in the treated gas close to 4 ppmv. Fig. 6a shows that for the conditions of this specific case, TEG contamination has minimal effect on both CO 2 slip and H 2 S removal despite the total acid gas loading doubling. However, it may be noteworthy that substantial contamination by TEG actually slightly improves H 2 S removal and, although not shown here, 50 wt-% contaminated MDEA shows a slightly higher temperature bulge (54.2°C vs. 50.3°C).
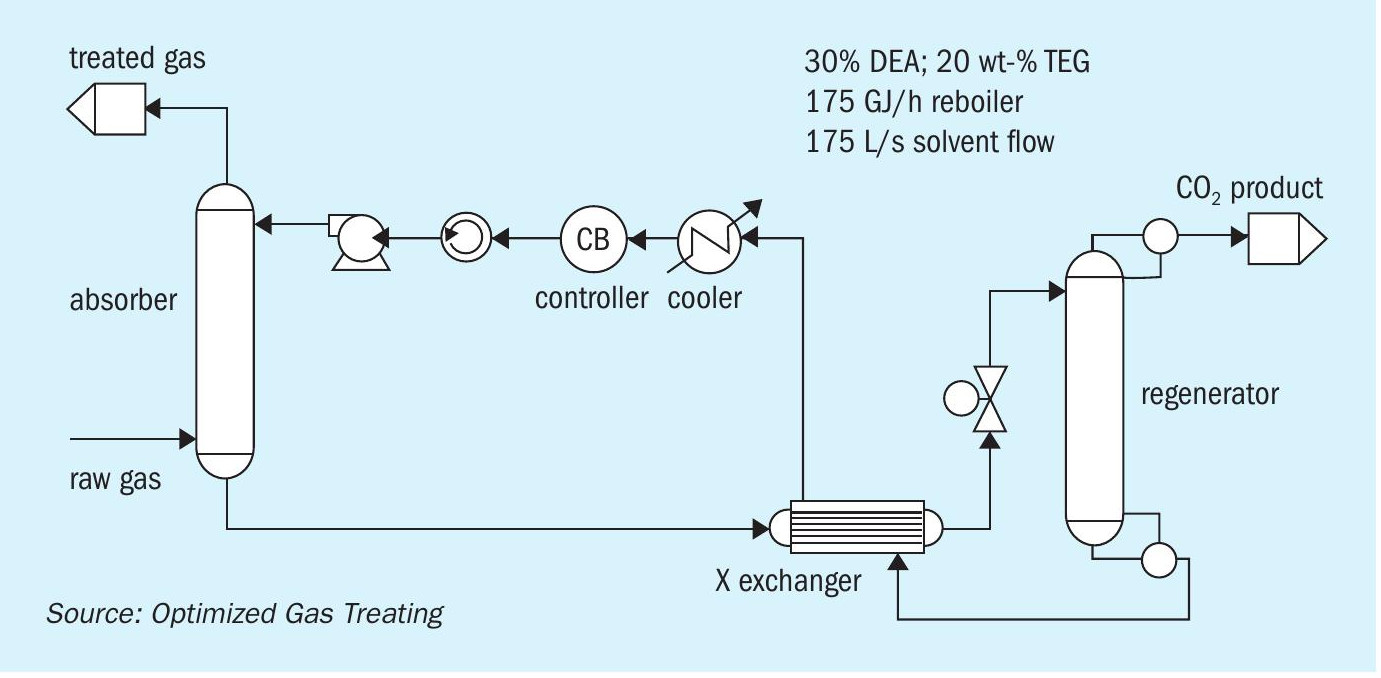
DEA
The TEG-free case was run under conditions that produced about 500 ppmv CO 2 (ammonia syngas quality) but lowered the H 2 S content to about 2 ppmv. As the TEG level increases (Fig. 6b) a point is reached where the treating becomes determined not by solvent lean loading but by solvent capacity for absorbing acid gas. This kind of behaviour will occur for any amine because each has limited capacity. The initial improvement in H 2 S removal results from improved VLE whereas with CO 2 the VLE improvement is insufficient to counterbalance the effect of TEG and CO 2 loading on viscosity.
pMDEA
With pMDEA, increased contamination for the case shown in Fig. 6c leads to enhanced H 2 S removal and increased CO 2 slip. The H 2 S content of the treated gas is completely determined by the vapour-liquid equilibrium because, at and below 47.5 wt-% TEG, the absorber is completely lean-end pinched with respect to H 2 S absorption. This is illustrated in Fig. 7 which shows the actual and equilibrium H 2 S partial pressure profiles for 47.5 wt-% merge at the top of the absorber (no driving force for absorption) but remain separated for 50 wt-% TEG. The 50 wt-% TEG case is mass transfer rate limited. Lower TEG levels are equilibrium limited so the effect of TEG on the VLE sets the level of treating for H 2 S. It should be noted, however, that in a mixed gas system such as this one, CO 2 absorption has a direct effect on H 2 S phase equilibrium.
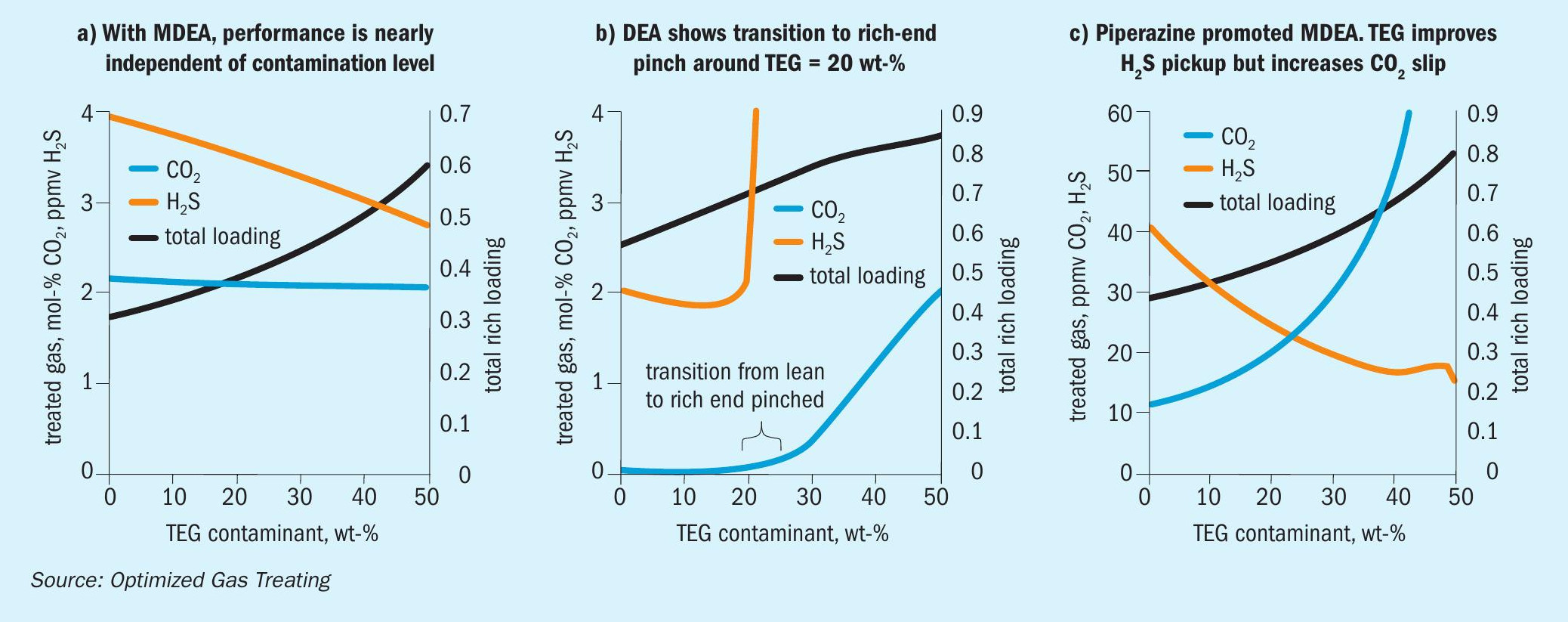
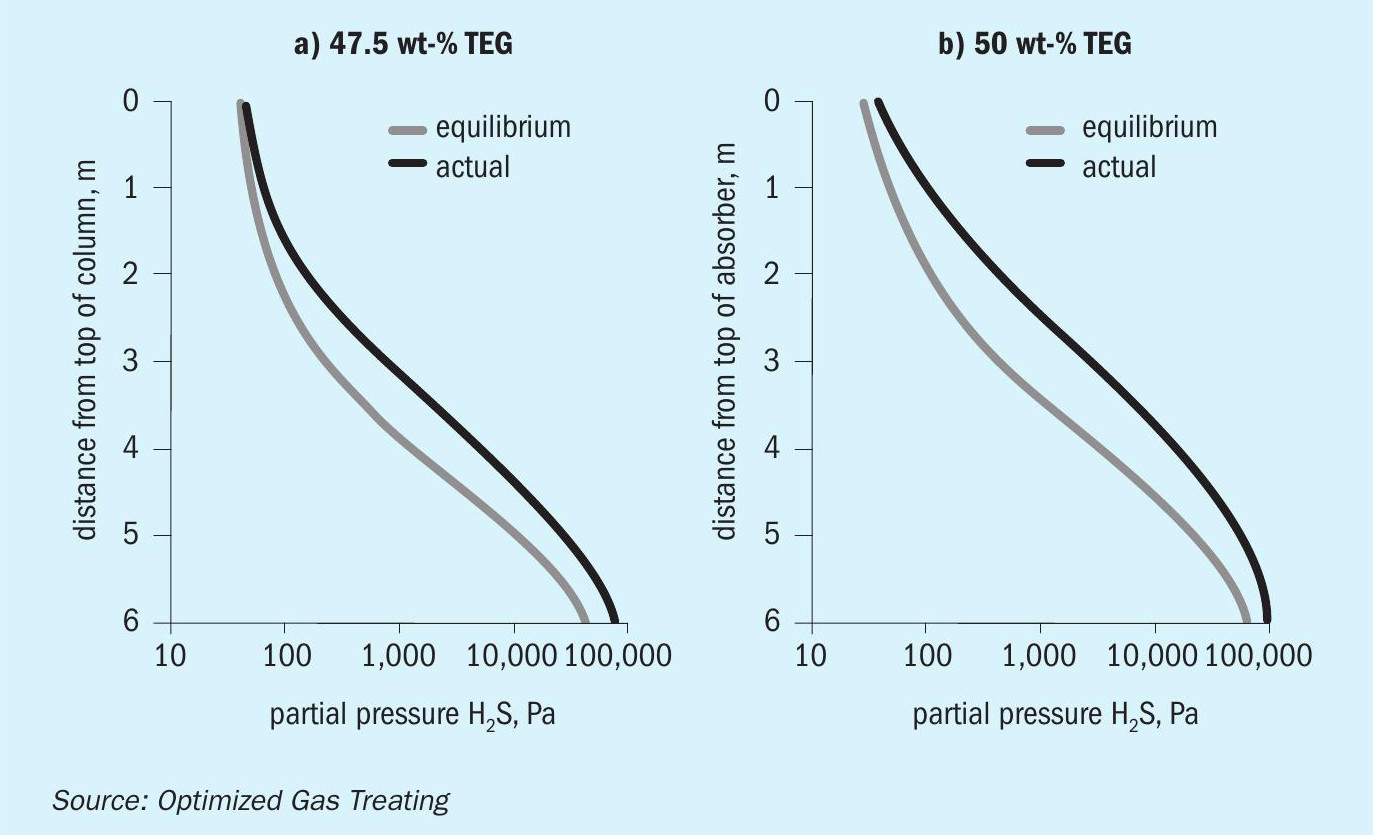
Summary
The effect of glycol contamination of amines on phase equilibrium and treating performance can be readily assessed by considering the contaminated amine as a hybrid solvent with glycol the inert component, and by using OGT | ProTreat’s hybrid solvent simulation capability to do the analysis.
OGT | ProTreat ® now offers a mass transfer rate-based simulation capability for hybrid solvents. The thermodynamic model uses a rigorous activity coefficient basis and develops the ternary and quaternary solvent description using mixing rules based on sound thermodynamics. Mass transfer rate calculations use the same proven and well-accepted rate model as is used in the ProTreat simulator generally. The result is highly reliable, predictive simulation of hybrid solvents. The non-reactive hybridising component in this case is TEG which is shown to increase somewhat the solubility of the acid gases in the hybrid. The effect offers a slight benefit to TEG contaminated amines compared with their virgin counterparts. ProTreat is now in a position to simulate sulfolane-based and TEG-contaminated hybrid solvents using a generalised framework that permits other hybrids to be simulated as well.
References