Sulphur 408 Sept-Oct 2023
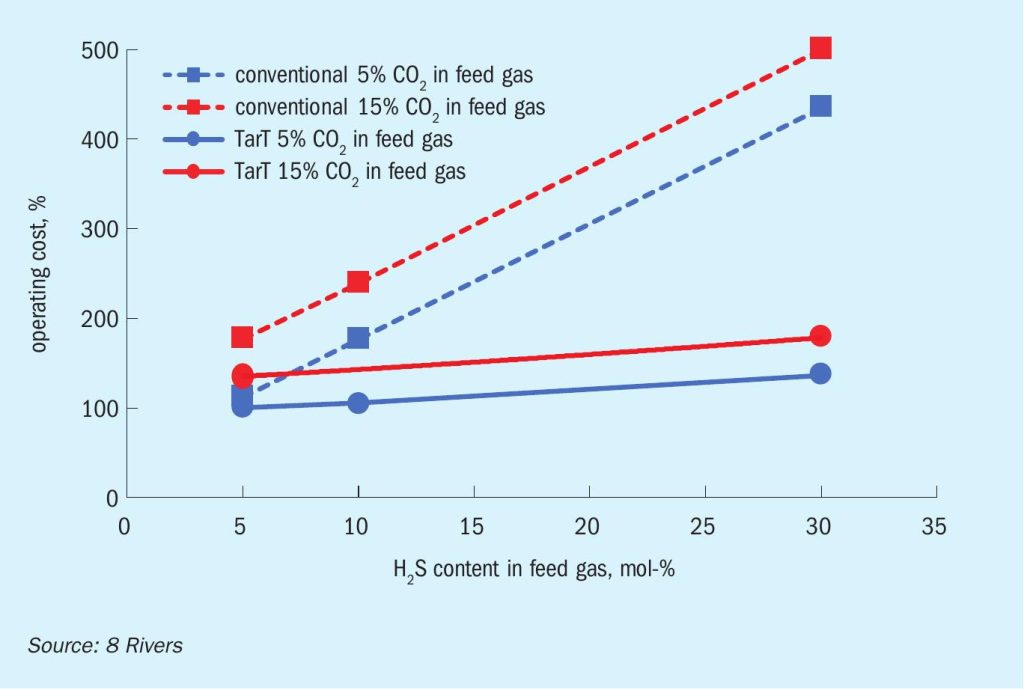
30 September 2023
Unlocking the power of sour gas reserves
DECARBONISATION
Unlocking the power of sour gas reserves
TarT technology, one of 8 Rivers’ decarbonisation technologies, shows promise as an economical, efficient, sour gas sweetening process with near-zero carbon dioxide emissions, and may be key to unlocking access to the world’s sour gas reserves.
Gigaton-scale, affordable, and decarbonised solutions are needed to support the world’s growing energy demand while facing our climate crisis. To avert the climate crisis, technologies that can meet the scale and access needs while averting billions of tonnes of carbon dioxide (CO2 ) not thousands, will create a key bridge to the energy transition.
It is essential that we explore and adopt pragmatic, affordable, and immediate solutions to make the 2050 net-zero emissions goal set by the United Nations Coalition1 realistic, as a delay in adopting these solutions only adds to future challenges. One such opportunity is the need to scale ultra-low carbon natural gas. As a displacement to coal, greater access to ultra-low-carbon natural gas can reduce global emissions by over 15%.
Natural gas as a decarbonisation pathway
Under current market conditions, natural gas is the most effective gigaton-scale fossil fuel-based solution available today. Per an IEA report, 98% of natural gas consumption has a lower emissions intensity than coal in power or heat applications2 . Switching from coal to gas is expected to reduce greenhouse gas emissions by 50% when producing power and 33% when producing heat. Across the developed world, the shift is already underway: as coal plants retire (in many cases, earlier than originally scheduled), utilities replace them with a combination of gas and renewables. In the developing world, where governments’ first priority is expanding energy access, the battle is less over existing coal-fired power plants than planned ones. As cost-conscious developing countries industrialise, meeting socio-economic growth and decarbonisation plans will depend on delivering the economic case that gas is the more affordable option to prevent future coal infrastructure from construction.
Whether decarbonisation plans include retiring existing coal plants or averting the construction of new ones, cost, access, and security of energy will be at the centre of an effective and equitable energy plan. Countries that have taken advantage of low-cost fuels and energy to grow their economies have the ability to invest in more speculative options to decarbonise. Developing countries must regularly weigh decarbonisation against fundamental infrastructure pillars, such as education, healthcare, and energy investments. Furthermore, while developed countries bear the brunt of the impact from historical emissions, it is the economic growth and stability of developing economies that will shape 21st-century emissions. Unlike the expensive energy storage systems needed to make renewables dispatchable, natural gas can deliver reliable baseload power with tremendous emissions reductions in a manner affordable without subsidies.
Decarbonisation case study: The American (shale) revolution
Recent history underlines that reductions in natural gas pricing can deliver significant emissions impact. In 2008, innovations in fracking technology unlocked large swathes of previously inaccessible United States gas reserves. As US access to proximate, abundant, affordably-produced natural gas grew, prices fell. And as prices fell, so did the carbon emissions.
Between 2005 and 2021, US carbon emissions fell even as the nation’s overall energy consumption rose (see Fig. 1). Even as state and federal governments, locked in partisan gridlock, struggled to enact climate policy, its emissions reductions soared past those of far “greener” Germany, as well as Japan and the UK. Meanwhile, rapidly industrialising China saw its emissions skyrocket as it built out its coal capacity, even as it increased its renewables investments3 .
The fact that areas with lower natural gas prices saw emissions drop significantly despite greater electricity generation, while areas with high natural gas prices saw the reverse, suggests that lowering the cost of natural gas is a key short-term decarbonisation lever.
To catalyse a similar scale of cost (and, ultimately, emissions) reductions worldwide, we need a similar stimulant to access underutilised natural gas supplies. Thankfully, the pathway to access this resource is straightforward: more efficient sour gas processing with inherent carbon capture.
Sour gas processing: the next “revolution” in natural gas access
More than 40% of the world’s natural gas reserves contain enough sulphuric contaminants to qualify as sour, but processing it poses operational complexity that affects the final product’s cost intensity4 . These high costs, combined with other technical hurdles, render billions of cubic feet of sour gas impractical globally. Consequently, a technology that can sweeten gas more economically could have a game-changing impact on the next several decades of emissions reductions by reducing the cost of natural gas for those deposits.
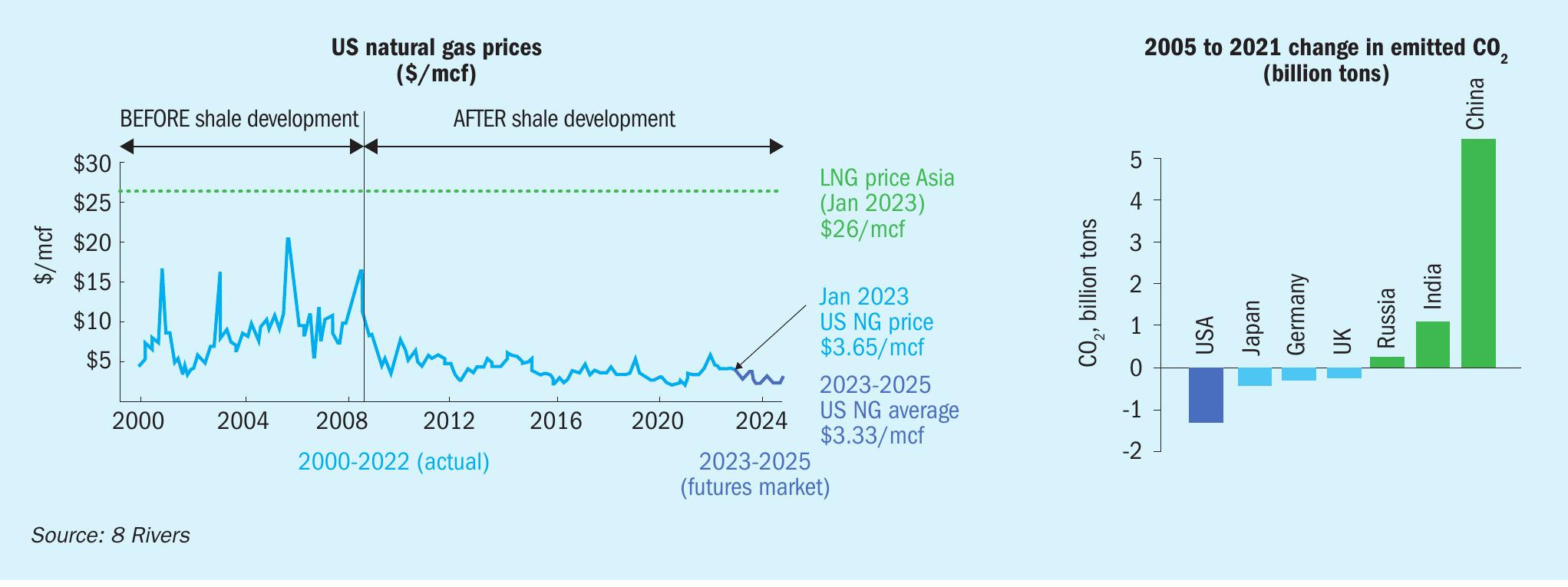
Historically, sour gas processing has faced economic and environmental barriers. On the economic side, challenges include high processing cost, high cost of acid gas reinjection, poor scalability, and an oversupplied elemental sulphur market. On the safety and environmental side, challenges include H2 S toxicity, high carbon intensity, harmful SO2 emissions, and difficulties around carbon capture integration presented by conventional processing technologies.
Despite these ongoing challenges, the sour gas processing industry has been static. “Most innovations I’ve seen in recent decades have been associated with incremental improvements in the conventional sour gas treating process, and new types of sulphur recovery tail gas treating processes to drive regulation-mandated efficiency gains,” says Angela Slavens, Managing Director of UniverSUL Consulting. “For example, when I started my career, Sulphur recovery units needed 99% efficiency. Now, tail gas treating requirements demand >99.9% efficiency, so we’ve seen technologies respond.” But those gains stemmed mostly from regulation, not from a perceived market need.
However, a changing environment (figuratively and literally) means the industry is poised for a shakeup. “Recently, the industry is changing so much [thanks to] the move toward decarbonisation. [It] is definitely changing the way people look at new opportunities. I’ve seen a lot more appetite for new technology. Whether organisations move quickly on it, I don’t know. But I think people will be most interested in new technologies proven to save money, reduce carbon footprint, and demonstrate staying power throughout the energy transition,” Ms. Slavens says.
Highly sour processing capability can secure sulphur’s future
The industry’s increased emphasis on efficiency isn’t only for decarbonisation. It’s also to increase access to sulphur in a future where natural gas may, eventually, pose a lower proportion of the overall energy mix. Ms. Slavens says the future may well incentivise seeking out extremely sour wells to ensure sulphur access: “For example, in the early 1990s, when sulphur was briefly in short supply, Shell tapped an Alberta gas reserve with over 90% H2 S content to prove that they could extract high levels of sulphur from such wells if needed.”
Eventually, Ms. Slavens predicts, such a need may arise – meaning that the sour gas processing “technology of the future” must not only be lower-emissions and more efficient but able to handle extremely high levels of H2 S. “Since the start of the shale gas revolution in the US, I’ve been speculating about when we may eventually need to prioritise such highly sour gas to meet sulphur demand, though no one knows on what timeline. I just hope it happens before I retire. If not, I’ll have to come out of retirement, just to see sulphur having its heyday,” she laughs.
Twofold need for processing innovation
Overall, the case is clear: economic sweetening of highly sour natural gas is important for two reasons. First, it advances decarbonisation by providing cheaper natural gas, which can then accelerate the transition away from coal. And second, it helps to secure the future supply of sulphur, an ingredient in key industrial products from fertilizers to pharmaceuticals. And after years of seeing only small modifications to sour gas processing technology, Ms. Slavens thinks the appetite for change is here: “A sour gas sweetener that operates more efficiently with lower emissions would make a huge difference to the industry,” she says.
Indeed, 8 Rivers’ forecasts estimate that reducing the cost of sweetening could achieve significant impact, and has developed its TarT technology to deliver these economic and decarbonisation gains.
TarT: ultra-economical sour gas processing with inherent carbon capture
8 Rivers has designed a cryogenic sour gas sweetening technology, TarT, that achieves both efficiency gains and emissions reduction.
TarT originated at a conference in the Middle East after 8 Rivers presented NET Power, an 8 Rivers-born legal entity to market the Allam-Fetvedt cycle for carbon-free power generation using natural gas. Attending energy organisations, mindful of the Middle East’s magnitude of sour gas, asked whether the zero-emissions power cycle would work with high H2 S content. At the time, the answer was no. But 8 Rivers’ culture of entrepreneurship and ingenuity wouldn’t let the answer stay “no” for long. After a company-wide competition, TarT emerged – an affordable, zero-emissions sour gas processing solution compatible with the zero-emissions Allam-Fetvedt power cycle and other systems that utilise natural gas as a feedstock or fuel, so long as any resulting CO2 is captured from its utilisation.
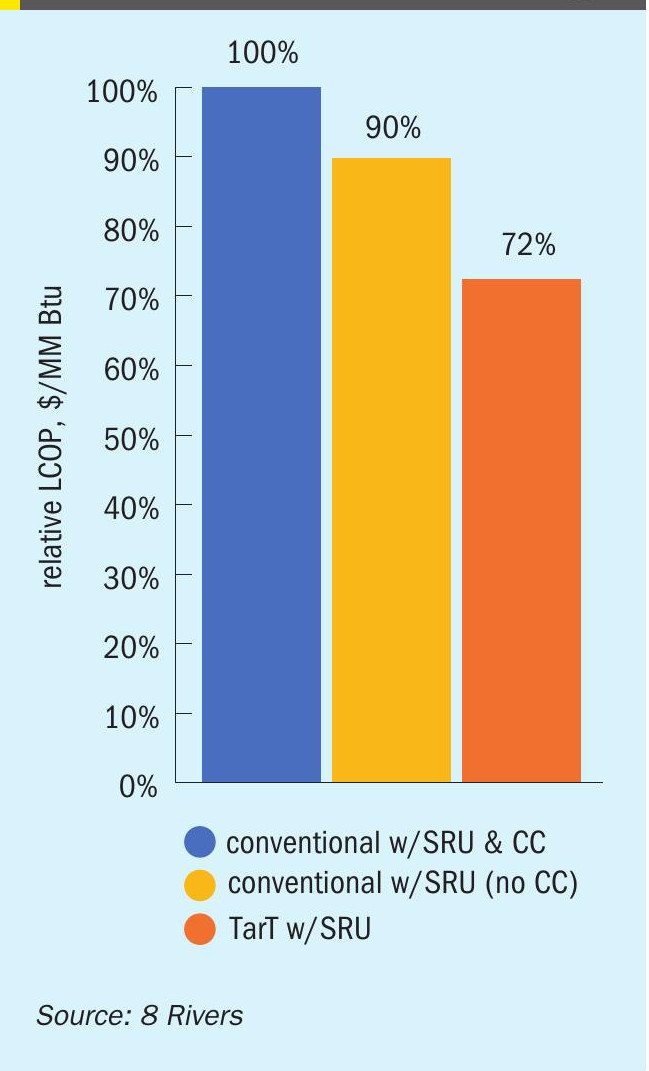
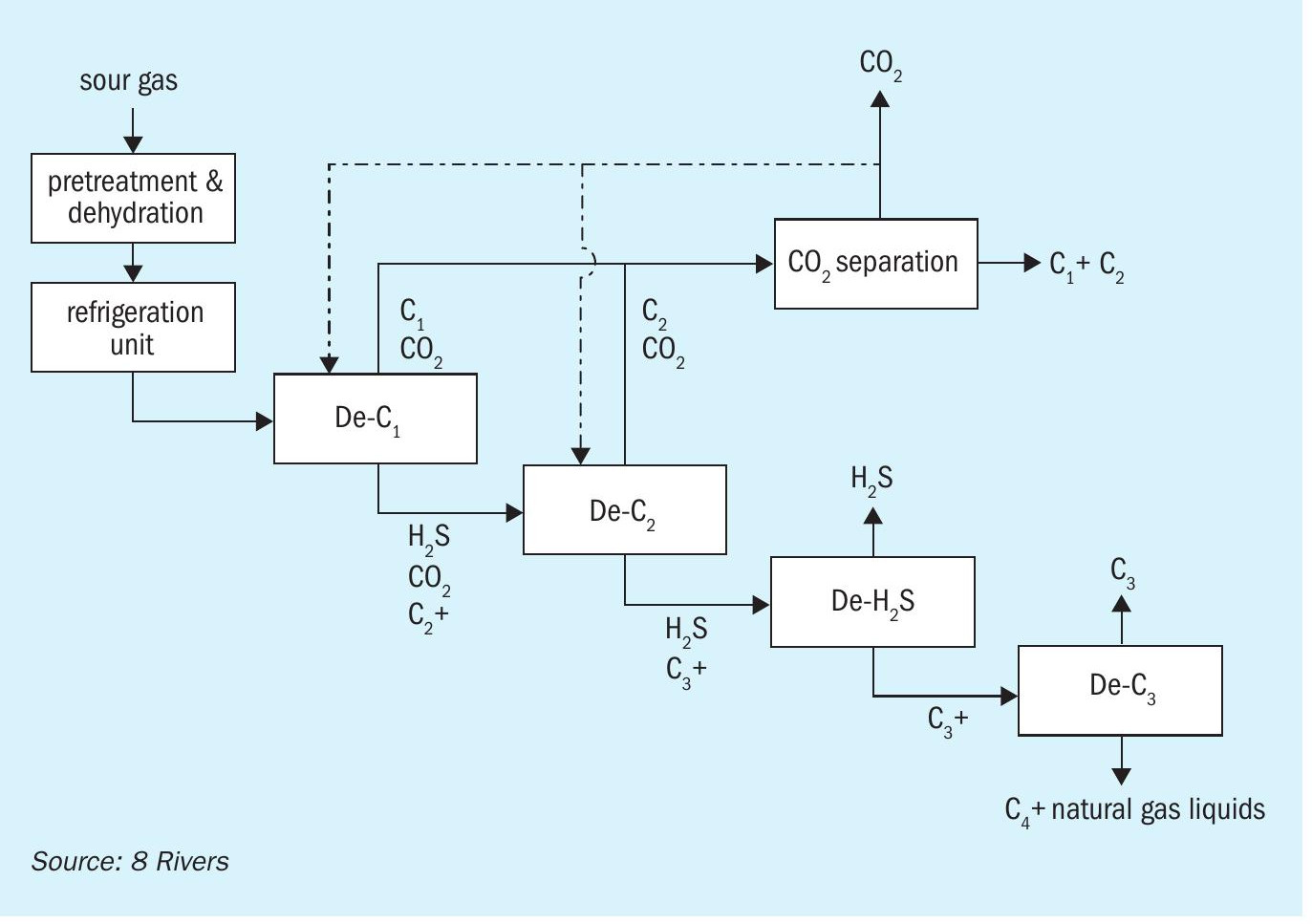
TarT deploys liquid carbon dioxide’s properties as a solvent to achieve bestin-class efficiency and inherent carbon capture. While engineers may find it counterintuitive to deploy a contaminant as the first step in a purification process, the carbon dioxide input is key to TarT’s success.
TarT processes sour gas high in H2 S at lower costs than conventional amine-based technologies, with none of their associated environmental concerns, such as emission of volatile organic compounds (VOCs). Furthermore, TarT’s inherent carbon capture makes it even more cost-competitive when compared with conventional amine processes paired with costly, inefficient backend carbon capture technologies. A case study was performed by 8 Rivers comparing TarT to a conventional amine-based sweetening process with carbon capture and natural gas liquid (NGL) recovery. The feed gas studied had an H2 S and CO2 content of 16 and 8 mole percent, respectively. TarT was demonstrated to have a 28% reduction in its levelised cost of sweetened natural gas production (LCOP) relative to the conventional amine process with a carbon capture retrofit (see Fig. 2). The TarT technology displayed an 18% reduction in natural gas LCOP relative to the conventional process with no carbon capture as well. This improvement is displayed in Fig. 2. The amine used in this study was DEA and a sulphur recovery unit was included for both technologies. The TarT process inherently performs NGL recovery and carbon capture (CC), so these units had to be added to the conventional technology to perform a uniform comparison.
Additional benefits include a modular, skid-mounted design that can be easily scaled up or down, making it more feasible for deployment to small, remote reserves that might otherwise be stranded. Recent announcements by governments can further incentivise and facilitate investment into concurrent H2 S and CO2 technologies, such as TarT, compared to other less efficient carbon removal technologies.
Regardless of these incentives, the best way to understand TarT’s standout effectiveness is to examine its process and emergent features in more detail.
Process overview
By reimagining what others see as a waste stream to see its potential as a feedstock, TarT integrates sour gas sweetening with carbon capture, NGL recovery, and acid gas enrichment. TarT’s cryogenic distillation system leverages liquid CO2 ’s properties as a natural solvent to enable inherent CO2 capture. Where other technologies vent sour gas’s embedded carbon dioxide to the atmosphere, TarT captures it for sequestration or utilisation. The resulting low-CO2 sales gas (<2 vol-%) can qualify as “low-carbon” natural gas, making it a cost-effective feedstock for clean ammonia synthesis, especially when paired with 8 Rivers’ ultra-low-emissions hydrogen production technology,8 RH2 . And importantly, TarT has no upper limit for H2 S and CO2 content. So if Ms. Slavens’ “drilling for sulphur” hypothetical does come to pass, TarT will be up to the challenge.
Fig. 3 shows the main process steps for the base configuration of the TarT process in which pipeline quality natural gas, LPG, C4 + NGL blend, and high-pressure high-purity H2 S and CO2 streams are generated. There is an option to run the process in a C2 recovery configuration, generating a pure ethane stream. This requires a few additional process steps. By looking at the arrangement of equipment in Fig. 3, one can conclude that the TarT process relies on equipment that is all commercially available. In fact, there are several decades of experience in the oil, gas, and petrochemical industries that can be beneficially used to design a TarT sweetening and NGL recovery plant. The functionalities of the main process steps for the base configuration of the TarT process (Fig. 3) are described in Table 1.
The coldest sections of the plant in the base case configuration of the TarT process are the overhead condensers in the De-C1 and De-CO2 towers, which are nominally set to -55°C. This provision minimises the risk of the formation of solid CO2 and H2 S during transient and steady-state operations that may otherwise occur in other similar low-temperature natural gas treatment processes, which tend to operate at temperatures below the triple point of CO2 at -56.6°C. In addition, it also eliminates the need of using costly equipment such as that employed in the controlled freeze zone (CFZ) process5 . Furthermore, the use of a membrane for additional CO2 removal eliminates the need to generate the colder temperatures, around -90°C, which are required in some other low-temperature techniques, as well as the much higher thermodynamic inefficiencies of a refrigeration loop at such low temperatures6 .
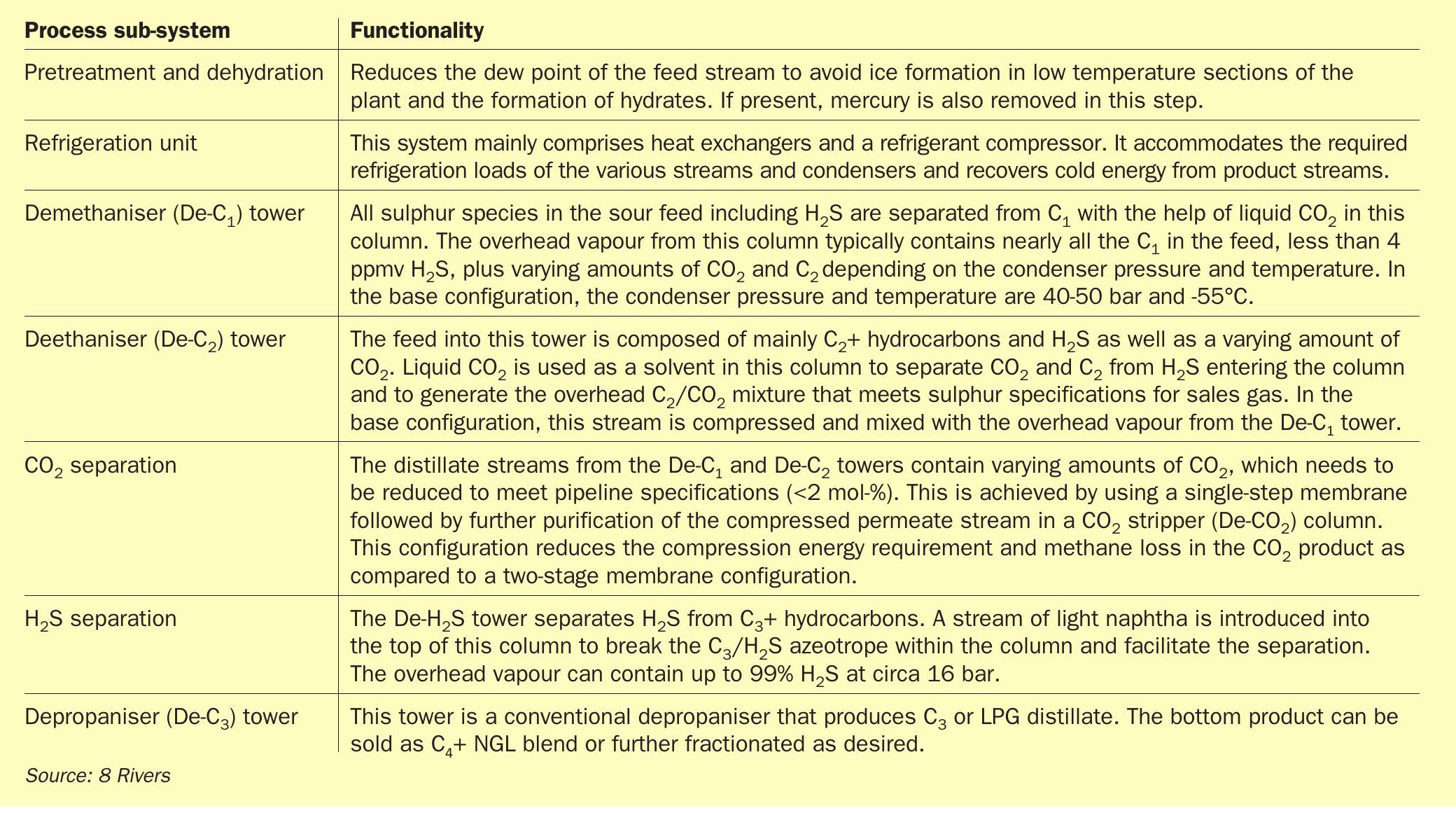
The TarT process also resolves one of the main challenges in the low temperature separation of sour gas species by using liquid CO2 to enhance the separation of C2 from H2 S in a De-C2 column and to maximise C2 recovery from such processes. Historically, when C2 is available in the sour gas and a low-temperature process is used for CO2 separation, H2 S eventually ends up with the C2 and an additional step of H2 S separation from the C2 stream is required. This leads to additional processing costs and typically results in low C2 recovery, or to choosing conventional sweetening processes such as amine-based technologies and its associated cost and energy efficiency penalties.
In practice, when a high-pressure CO2 product for enhanced oil recovery or sequestration is desired from a gas processing plant, the maximum allowable H2 S in the CO2 product may be as low as 10 ppmv. Using the conventional amine process, this would require an additional amine train for selective removal of H2 S from either sour gas or the resulting CO2 /H2 S mixture from the first amine unit. For example, DEA solvent can be used in the first amine unit for selective removal of H2 S from sour gas with minimum CO2 co-absorption followed by a DGA amine unit to remove the remaining CO2 in the sour gas. This CO2 can be dehydrated and pressurised for EOR application or sequestration. This configuration generally increases both the capital and operating cost of the conventional technology option, as demonstrated in Fig. 2. In contrast, the TarT process inherently generates separate CO2 and H2 S streams with high purities and at desired pressures. The resulting high-pressure H2 S stream can be converted to a value-added sulphur product or safely and permanently sequestered in a depleted oil/gas field or a permeable saline aquifer.
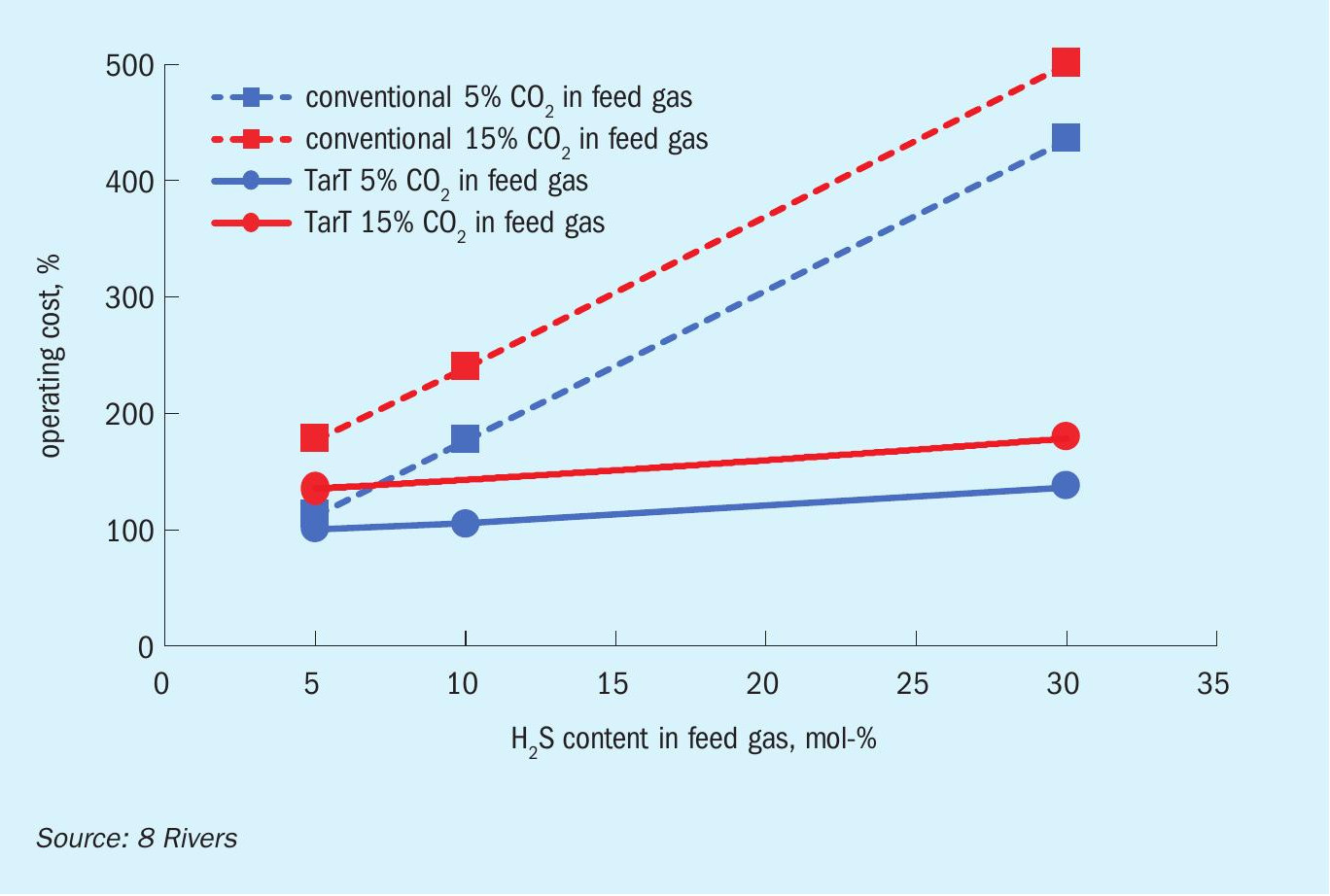
The TarT technology has a very flexible process configuration that allows for optimisation based on the composition of the sour gas feed, desired product output, market conditions in the relevant jurisdiction of deployment, and the specification and characteristics of the site. It economically sweetens even the sourest natural gas reserves, captures the natural CO2 present in the gas, enriches the H2 S, and recovers the natural gas liquids.
Opex-advantaged with highly sour gas
TarT enables access to gas reserves whose acid gas content would be economically inaccessible with conventional amine technologies. Plus, its edge in opex advantage over amine-based sweetening increases in tandem with H2 S content (see Fig. 4). And, while not included in the chart in Fig. 4, TarT is even more cost-effective once you factor in the price of carbon capture, for operators who choose it, on conventional amine technologies.
Deployable to remote fields
But TarT’s reduced sensitivity to H2 S content in feedstocks is not the only way it makes “economically inaccessible” reserves accessible. Its modular construction enables each block to be designed and built independently, which drives down capex due to economies of scale and learning effects. Thanks to its more flexible size, TarT can also operate more economically at smaller scales than conventional processes, a feature which boosts its ability to handle small, remote reserves that might otherwise be stranded assets.
Conclusion: Unlocking decarbonised fuels through unconventional thinking
While “purification by contamination” may not be a strategy in engineering textbooks, the resulting savings in cost and carbon attest to the value of thinking outside of the box. To meet mid-century decarbonisation goals, the energy industry will require much more innovative thinking. It is imperative not only to dream up futuristic, “moonshot” solutions but to reimagine practical ways to decarbonise by deploying the technology already on hand.
Given natural gas’s potential to accelerate global decarbonisation, and the proportion of sour gas in the world’s gas reserves, the sulphur industry will be an integral player in the fight to achieve emissions reductions by replacing coal with gas. 8 Rivers looks forward to collaborating with sour gas processors and the broader sulphur industry in this endeavour.
References