Nitrogen+Syngas 386 Nov-Dec 2023
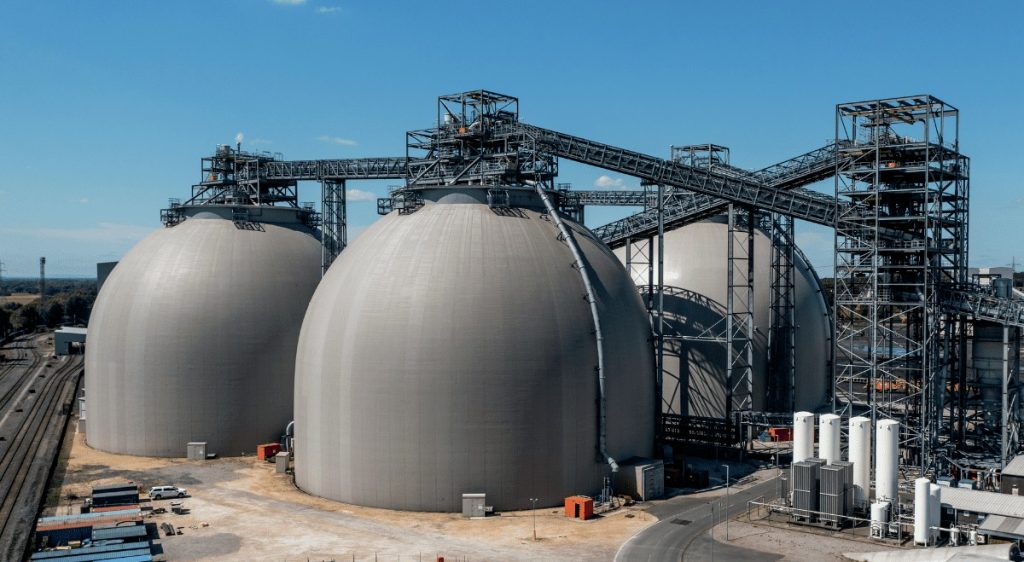
30 November 2023
Constraints on renewable production
ENVIRONMENT
Constraints on renewable production
With low carbon ammonia and methanol being considered not just for their chemical and fertilizer uses, but as fuels, can we make enough of them to fill our energy needs?
Low carbon hydrogen is increasingly being seen as a route to lower carbon production of the fuels and chemicals that our civilisation depends upon. And the most prominent among the routes to these low carbon fuels and chemical feedstocks rely upon the two main current uses for hydrogen; ammonia and methanol production. The global ammonia market currently stands at around 180 million t/a, representing 30 million t/a of hydrogen production by weight, and the global methanol market is around 100 million t/a, representing another 12.5 million t/a of hydrogen. While these figures are certainly impressive, however, fuels markets in particular are of an order of magnitude larger. There is therefore a question as to whether it will be sustainable to base fuel and chemical production upon low carbon ammonia and methanol, and whether sufficient renewable energy resources can be tapped to generate them.
Ammonia
At the moment, ammonia is mostly (ca 80%) used as a feedstock for fertilizer production, with most of the rest going to produce various industrial chemicals such as melamine, caprolactam, etc. It is unlikely that these markets will increase drastically over the coming years, with growth tending to be incremental. In the field of nitrogen fertilizers, there are attempts to correct overapplication and leaching into water courses and to reuse nutrient bearing material flows which may indeed reduce consumption in the longer term, or at least balance increased consumption in regions such as Africa which currently under-apply fertilizer.
However, new uses for ammonia have the potential to dramatically increase consumption over the next 20-30 years. There is serious consideration being given to ammonia use as a maritime fuel in order to meet upcoming International Maritime Organisation rules on carbon emissions. Maritime engine manufacturers are actively developing and aim to soon commercialise ammonia-fuelled two-stroke and four-stroke engines in the next few years for both new builds and retrofits, and believe that they can deliver commercial performance within existing regulatory limits for nitrogen oxides. There is also consideration being given to ammonia as a fuel for stationary power, particularly in Japan, where there is interest in burning low carbon ammonia in coal-fired power stations to lower emissions. JERA is demonstrating co-combustion of 20% ammonia and 80% coal in a 1 GW power plant, and the Japanese government roadmap targets the use of 30 million t/a of fuel ammonia by 2050, starting with co-combustion technologies and eventually moving to 100% ammonia combustion.
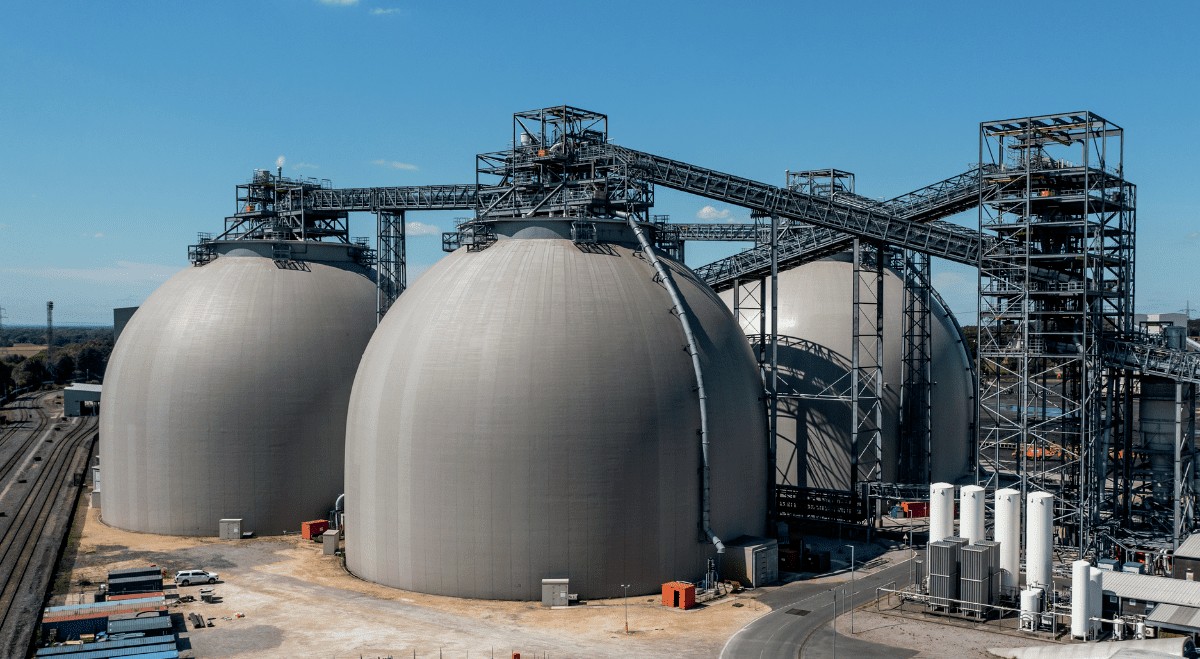
Ammonia is also proposed as a hydrogen carrier, to overcome the storage and distribution challenges of hydrogen, producing ammonia using low carbon hydrogen in regions where abundant solar or wind energy is available, and then ‘cracking’ it back to hydrogen and nitrogen where it is required.
The International Renewable Energy Agency (IRENA) has suggested in a recent report that global ammonia demand could increases from 183 million t/a in 2020 to 688 million t/a in 2050, including 230 million t/a of ammonia as fuel and 130 million t/a of ammonia as a hydrogen carrier.
Methanol
Methanol has already to some extent made the transition from a purely chemical feedstock to being used in fuel derivatives and even as a way to produce plastic precursor chemicals. Of the approximately 100 million t/a of methanol produced globally, around half is used as a feedstock for traditional chemicals production, including formaldehyde, acetic acid, methyl methacrylate etc. Another 20% is used to make olefins (MTO), mainly in China. And the remaining 30% goes to various fuel end uses, including direct blending into gasoline, direct conversion of methanol to gasoline (MTG), esters which are used as gasoline additives, esterification of vegetable oils for biodiesel production, and manufacture of dimethyl ether (DME) which is used as a blendstock in LPG.
However, as with ammonia, there is considerable interest in using low carbon methanol as a maritime fuel. Methanol has something of a head start on ammonia in that methanol marine engines have already been developed, and there are far fewer concerns in the shipping industry around safety as regards using methanol as a bunker fuel – ammonia by contrast is still viewed with some suspicion because of its toxic nature. Low carbon methanol has also been suggested for static power applications, and because of its versatile chemical nature could be used for production of low carbon plastics, gasoline etc. IRENA’s report on methanol, developed in conjunction with the Methanol Institute, suggested that by 2050, global methanol demand could have reached 500 million t/a.
Low carbon feedstocks
These figures; 688 million t/a of ammonia and 500 million t/a of methanol equate to 115 million t/a and 62 million t/a of low carbon hydrogen respectively. But how to generate that much low carbon hydrogen at scale is the question that must be answered before these projections can become a reality, especially when it is considered that they must be developed in parallel with a wholesale switch over from electricity generation via burning oil, gas and coal to renewables, itself taking place at the same time that electric vehicles are demanding more and more power from the grid. Is there enough renewable power available?
Carbon capture
Carbon capture and use/storage (CCUS) – so-called ‘blue’ ammonia or methanol – has been a favoured route for producing lower carbon production at scale, because it is able to make use of well proven technologies at relatively modest additional cost, at least compared to fully green production using electrolysis. The International Energy Agency (IEA) estimates that 15% of carbon reduction achieved by 2050 will need to use CCUS. However, development of CCUS has not been as fast as anticipated. Over the period 2009-2021, of dozens of planned projects, only 26 came to fruition, and all but six are used for enhanced oil recovery, where the oil revenues can balance the cost of implementation. Around 25 million t/a of CO2 is captured from these projects. It is estimated that on the basis of currently announced projects this could rise to 700 million t/a by 2050, but this is still far short of the 36 billion t/a of carbon dioxide that is currently being released to atmosphere each year, and only a fraction of the 6-7 billion t/a which the IEA projection says will therefore be required to be captured using CCUS in order to reach net zero. There is no shortage of available space in underground oil and gas formations, what is lacking is the financial incentive to press ahead with projects, though the US IRA has helped kickstart a number of new projects there by guaranteeing subsidies.
Nevertheless, a recent McKinsey report is more optimistic on the prospects for CCUS. It estimates that – given sufficient international cooperation and the availability of enough investment money – the development of 400 CCUS ‘hubs’ near large point source emitters (cement, power, steel, and chemicals such as ammonia and methanol) would be close enough to existing underground geological structures suitable for use in CCUS, and could capture a total of 9-10 billion t/a of CO2 at an average cost of $100/t, and could capture 4.2 billion t/a just using 110 hubs at an average cost of $85/t. Capture costs are lowest where carbon streams are already concentrated, and ammonia and methanol are particularly suited for this as they produce concentrated CO2 in process streams. It is also possible to use captured CO2 as a feedstock in renewable methanol production.
Biomass
Another route to low carbon production is to use biomass as a feedstock, usually waste from forestry or pulp and paper production, though municipal solid waste is now also lumped in with these. Conversion is via gasification. The issue for biomass is cost and scale. Gathering sufficient biomass to operate a large scale plant is a difficult and hence expensive task, and most plants that have operated have been based on small scale modular production. IRENA estimates the cost of ammonia production via biomass gasification to run from $450-2,000/tonne of ammonia, making it just about viable at the lower end of scale, but in its Renewable Ammonia Innovation Outlook, IRENA says that “biomass is not expected to play a major role in decarbonising ammonia production”.
Renewable electricity
Renewable electricity generation has been increasing rapidly, with 320 GW of capacity added in 2022 alone. High fossil fuel prices, the disruption to Europe’s energy supply caused by the war in Ukraine, and government policies such as REPowerEU in Europe and the IRA in the US have all played a part in the current surge in renewable installations. Total capacity is expected to reach 4,500 GW next year, equivalent to the combined generation capacities of the US and China, and 6,000 GW by 2027. Most of the growth is from solar and wind; suitable sites for new hydro-electric power are few and often contentious in terms of the land that is flooded.
Around 50-90 GW of the renewable electricity used by 2027 is earmarked for generation of green hydrogen, mostly for green ammonia and methanol production. This represents only around 2-4% of new renewable capacity. However, generating the 170 million t/a of clean hydrogen we discussed earlier will require 3,600 GW of renewable electricity capacity by 2050 at average solar/wind generation rates. While this will be well within the anticipated 20,000 GW of renewable capacity forecast to be installed by that time, it also requires the same amount of electrolyser capacity, which currently stands at just 0.6 GW worldwide. Capacity must therefore increase 6,000 times – an unprecedented, perhaps even impossible number. It is worth bearing in mind that installed solar power capacity has increased by a comparable amount over the past 25 years, but even so, this looks like a tall order, barring some technological breakthrough in the technology.
And of course costs will determine the rate of uptake, and here the news is less good. Although renewable power costs have come down dramatically, and electrolyser costs have fallen significantly, CRU estimates a current cost for green ammonia of $650-900/t, more than double that for blue ammonia. Carbon pricing and government subsidies can bridge some of that gap, and costs will continue to fall, but for the time being, that looks very expensive as something to be burned as a fuel; probably four times that of a liquid fossil fuel on an energy equivalent basis. The cost of green methanol is higher still, at around $750-1,200/t, though again blue methanol is much more comparable to existing bunker fuel costs. The conclusion for the ammonia and methanol industries would seem to be that blue is the way forward, at least for now.