Nitrogen+Syngas 388 Mar-Apr 2024
31 March 2024
Ultra-HP ammonia oxidation catalysts
NITRIC ACID CATALYSTS
Ultra-HP ammonia oxidation catalysts
Catalyst development for nitric acid plants is strongly dependent on operating pressures and nitrogen loading levels. Dr Hadi Nozari of Johnson Matthey provides a review of some key catalyst design principles, emphasising the critical role of operating pressure in catalyst selection and highlighting the innovative contributions of Johnson Matthey in advancing catalyst technology for ultra-high-pressure ammonia oxidation in nitric acid plants.
Tailored catalyst design derived from the plant-specific operating conditions is key for ensuring optimised performance of nitric acid plants. When it comes to efficiency, platinum-based knitted gauzes have been the catalyst of choice for production of nitric oxide, a precursor in the formation of nitric acid. These catalyst gauzes benefit from extensive research into ammonia oxidation kinetics and the dynamics of metal distribution within catalyst packs. Leveraging decades of expertise in catalyst design, Johnson Matthey has been offering the HICON™ corrugated catalyst, engineered specifically for ultra-highpressure (>10 barg) burners, to enhance catalytic performance.
Optimising ammonia oxidation
The performance of catalysts in ammonia oxidation fundamentally relies on the kinetics of the reaction. Ammonia reacts with oxygen through three main global reaction pathways, resulting in the formation of nitric oxide (NO), nitrogen (N2 ), and nitrous oxide (N2 O).
4NH3 + 5O2 4NO + 6H2 O ΔH0 = -907.28 kJ
4NH3 + 4O2 2N2 O + 6H2 O ΔH0 = -1104.9 kJ
4NH3 + 3O2 2N2 + 6H2 O ΔH0 = -1269.0 kJ
The selectivity to each product is largely determined by the catalyst composition and pack design. Different platinum alloys and pack layouts exhibit a range of nitric oxide selectivity. Plant operating conditions such as temperature and pressure also significantly affect the selectivity. At lower temperatures, nitrogen forms as the primary product, while higher temperatures and lower pressures favour nitric oxide formation. This temperature and pressure dependency comes from the differing surface chemistries involved in the reactions. For instance, at elevated temperatures, nitric oxide is less likely to react with ammonia on the catalyst surface, leading to increased selectivity to NO.
Industrial operating conditions typically fall within a temperature range of 850 to 940°C and pressure range of atmospheric to 13 barg, achieving nitric oxide selectivity between 90 to 97%. Based on the operating pressure and nitrogen loading ranges, the majority of nitric acid plants fall into one of the four categories in a general operational spectrum, as depicted in Fig. 1.
Achieving optimal selectivity for each plant type requires careful consideration of various catalyst parameters, including wire diameter, knit density, and layering. These physical characteristics influence the diffusion of reactants to the catalyst surface and thus impact reaction kinetics. Also, the catalyst’s metallurgical properties play a crucial role in determining its selectivity. Platinum, with its high selectivity to nitric oxide, is the preferred catalyst material for ammonia oxidation. Rhodium-platinum alloys, particularly those containing 3 to 5% rhodium, are commonly used due to their enhanced catalytic activity.
Ternary alloys of rhodium-platinumpalladium, containing high levels of palladium, demonstrate superior performance when placed in the lower half of catalyst packs in medium-pressure burners. These layers are considered more effective compared to conventional rhodium-platinum layers in this location. Leveraging these principles, Johnson Matthey’s ECO-CAT™ gauzes significantly reduce the required installed weight to achieve targeted nitric acid production levels, while increasing campaign length, improving conversion efficiency, and reducing nitrous oxide formation.
In addition to the primary reactions described above, secondary reactions may take place under certain conditions, where there is more residence time in the catalyst pack for remaining ammonia to react with nitric oxide to produce additional nitrogen and nitrous oxide.
4NH3 + 6NO 5N2 + 6H2 O
2NH3 + 8NO 5N2 O + 3H2 O
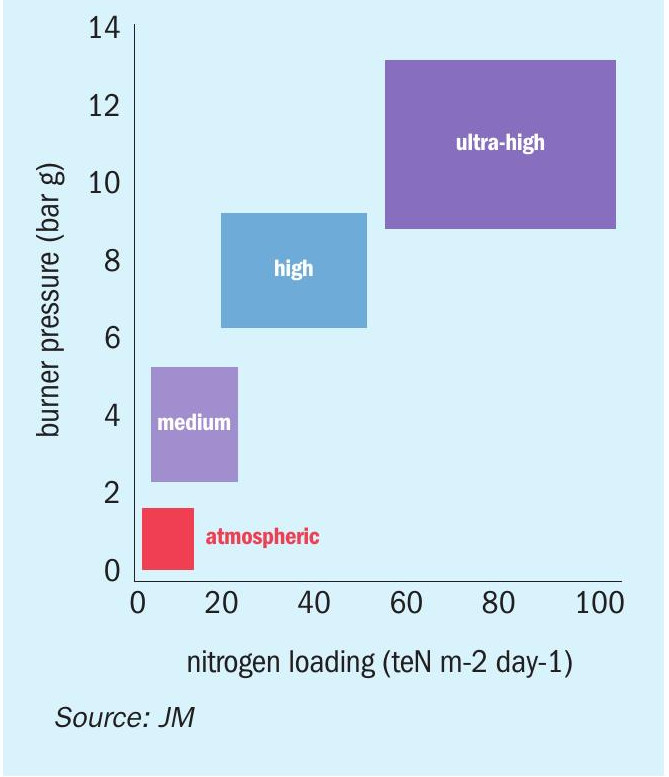
To mitigate these undesired reactions, it is important to ensure the completion of the oxidation reaction within a minimal number of catalyst layers, often achieved through use of denser knit structures, larger wire diameters or a combination of both at the top of the catalyst bed.
Understanding the intricate balance between the reaction kinetics, catalyst composition, and process conditions is critical for optimising selectivity and enhancing overall process efficiency in ammonia oxidation. Through continued research and innovation, Johnson Matthey strives to develop and optimise catalysts that meet the evolving demands of the nitric acid industry.
HICON for ultra-high-pressure oxidation
The operational features of ultra-highpressure nitric acid plants can significantly diverge from medium and low-pressure plants. Typically, ultra-high-pressure plants feature burner diameters of approximately 2,000 mm or less, with operating pressures reaching up to 14 bar and temperatures as high as 940°C. These conditions promote high gas velocities across the catalyst gauzes, resulting in shorter campaign lengths, lower conversion efficiencies, and higher metal losses. To address these challenges, Johnson Matthey has designed and developed the HICON (corrugated knitted catalyst), tailored to optimise conversion efficiency, pressure drop, light-off characteristics, and campaign lengths. Effectiveness of HICON in ultra-high-pressure plants relies mainly on increased surface area and the capacity to reduce gauze numbers while preserving installed weight.
The following provides a summary of several advantages of the HICON catalyst, along with the underlying physics that drive the enhancements.
Increased conversion efficiency
HICON boosts conversion efficiency by increasing the rate of gas film diffusion per unit cross-sectional area of the burner. This enhancement, as a direct result of reduction in superficial gas velocity, promotes the probability of gas molecules getting into contact with the catalyst surface. It is worth noting that Johnson Matthey has successfully combined HICON and ECO-CAT technology for ultra-high-pressure plants with very high loading (>90 teN m-2 d-1), resulting in further improvements in efficiency.
Faster light-off
The increased surface area of each gauze within the HICON catalyst pack increases the probability of ammonia-air mixture reacting on the catalyst surface. Essentially, this is an effective strategy to leverage fluid dynamics for achieving a faster light-off. A faster light-off significantly reduces the time required for the plant to reach the maximum efficiency. Subsequently, ammonia slip is minimised during the plant start-up.
Additionally, in cases where light-off is a significant problem, Johnson Matthey offers alternative proven technologies. These solutions involve increasing platinum concentration on the catalyst surface, effectively facilitating faster light-off.
Reduced pressure drop
Lower superficial gas velocity through the catalyst layers translates into proportional reduction of pressure drop across the catalyst bed. The pressure drop is lowered further by the reduction in the number of gauze layers in a HICON pack, compared to a standard pack of equivalent install pgm weight. This reduction in pressure drop boosts the likelihood of either increased production throughput or reduced energy consumption.
Considering that catchment pack has the largest contribution in pressure drop across the combined catalyst-catchment layers, lowering pressure drop across the catchment is highly advantageous. To address this, Johnson Matthey offers the A1-R, reduced pressure drop catchment, for ultra-high-pressure burners. A1-R, offered either flat or corrugated, features a higher porosity minimising pressure drop during the campaign.
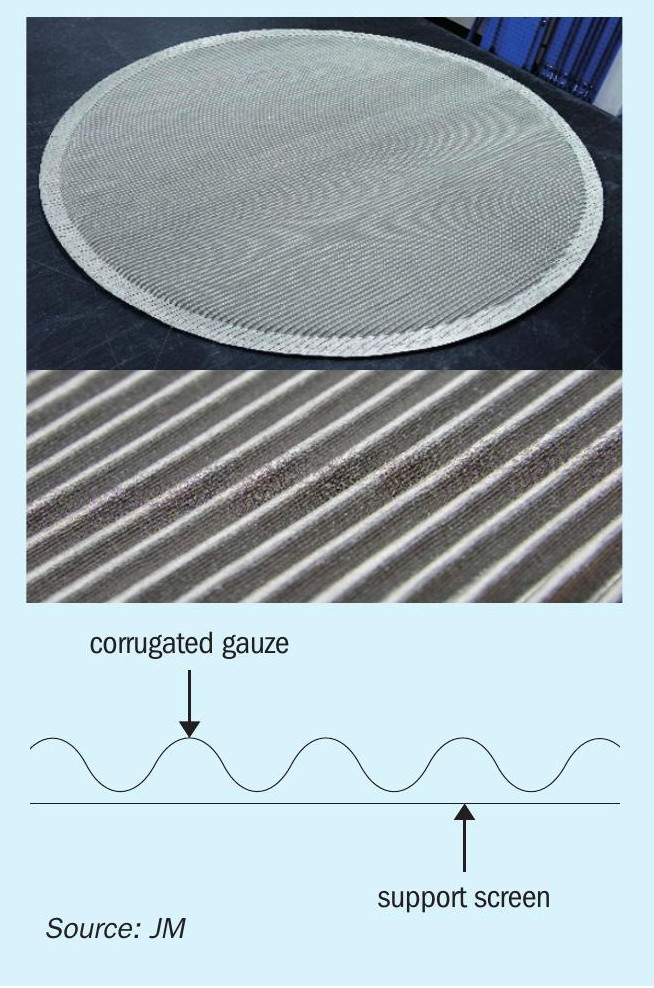
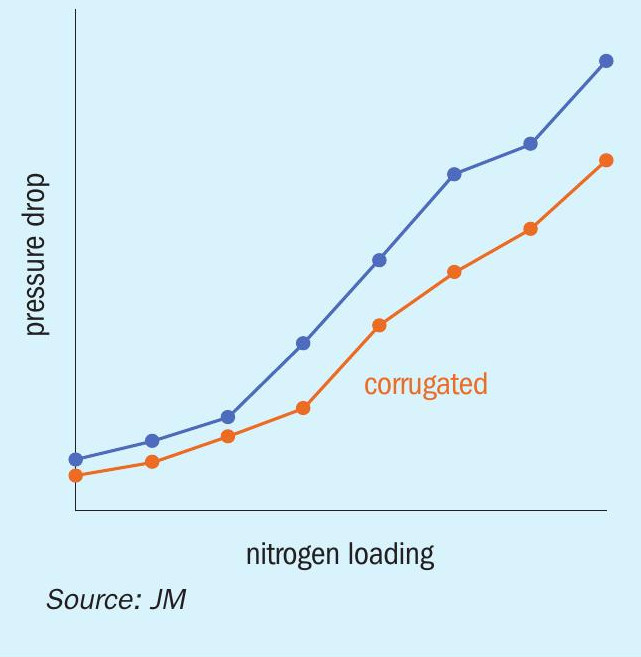
Extended campaign length
The rate of gross metal loss from the catalyst depends on operational parameters such as pressure, temperature, and gas stream velocity. By reducing superficial velocity, the HICON pack reduces the rate of gross burn-off, thereby prolonging catalyst life. Moreover, reduced metal loss mitigates the formation of rhodium oxide on the catalyst surface, preserving selectivity for ammonia conversion to nitric oxide while extending the catalyst life.
It is important to underline the significance of regular maintenance, particularly of air and ammonia/air filters, to sustain the maximum performance of the catalyst. Buildup of iron, silica, or aluminium will have a detrimental effect on catalyst life and operational efficiency.
Summary
This article explores some details of catalyst development, highlighting tailored solutions for ultra-high-pressure burners. In this framework JM’s HICON (corrugated knitted catalyst), is engineered to address the unique challenges of high-pressure oxidation environments. With improvements ranging from increased conversion efficiency to accelerated light-off, HICON represents a significant advancement in catalyst technology for ultra-high-pressure nitric acid production.