Fertilizer International 521 Jul-Aug 2024
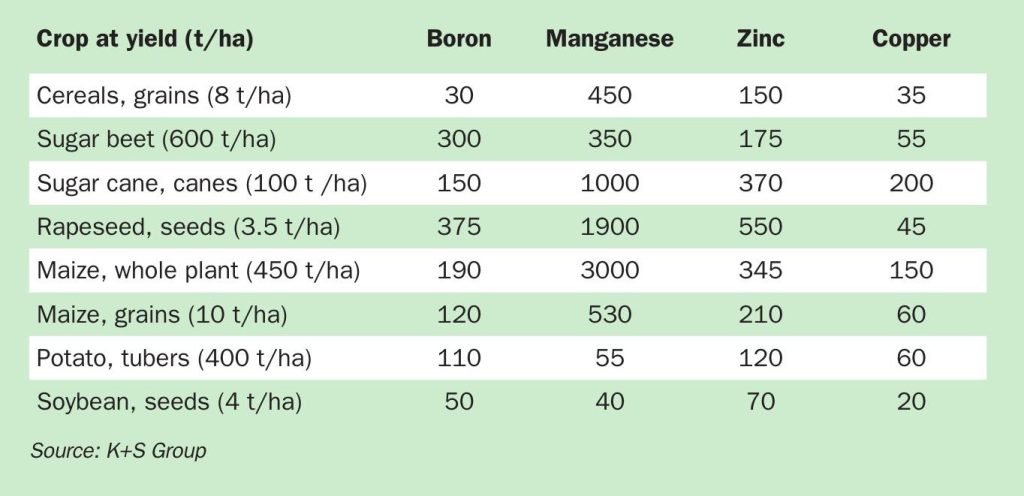
31 July 2024
Micronutrients – their unquestionable crop benefits
MICRONUTRIENTS
Micronutrients – their unquestionable crop benefits
Dr Setareh Jamali Jaghdani and professor Jóska Gerendás of K+S Group outline how micronutrient management, by positively influencing plant physiology and development, helps maximise crop yields.
Introduction
The need to secure high quality food in ever larger amounts has been an almost permanent challenge for global agriculture. Consequently, numerous studies since the 19th century have focussed on delivering greater crop yields by optimising plant nutrition 1 .
The increased application of commercial fertilizers since the late 1950s 2 has undoubtedly led to higher crop production. However, meeting growing food demand from an increasing world population still poses problems, especially with expectations that the world’s population will reach 9.2 billion by 2050 3 . To feed this rising population, there is a consensus that integrated water, soil, and nutrient management is necessary to ensure sustainable crop production 4 .
The 14 mineral nutrient elements which are vital for plant growth are divided into two groups: macro (major) nutrients and micronutrients 5 . Major nutrients are required in large quantities by plants (> 1 ‰ dry matter, DM) as they are ‘building block’ constituents of the macromolecules which form cell structures. Micronutrients, in contrast, are needed in smaller quantities (< 0.1 ‰ DM) and mostly activate plant enzymes. Still, this much lower plant requirement for micronutrients does not mean that they are less important. Indeed, crops cannot function properly and complete their growing cycle without them.
Micronutrients include the metal cations iron (Fe), manganese (Mn), zinc (Zn), copper (Cu), and nickel (Ni) and the anion-forming nutrients boron (B), chlorine (Cl), and molybdenum (Mo), listed in decreasing order of plant demand (Table 1). From a global perspective, the micronutrients Mn, Zn, and B are most relevant and are therefore the main focus of this article.
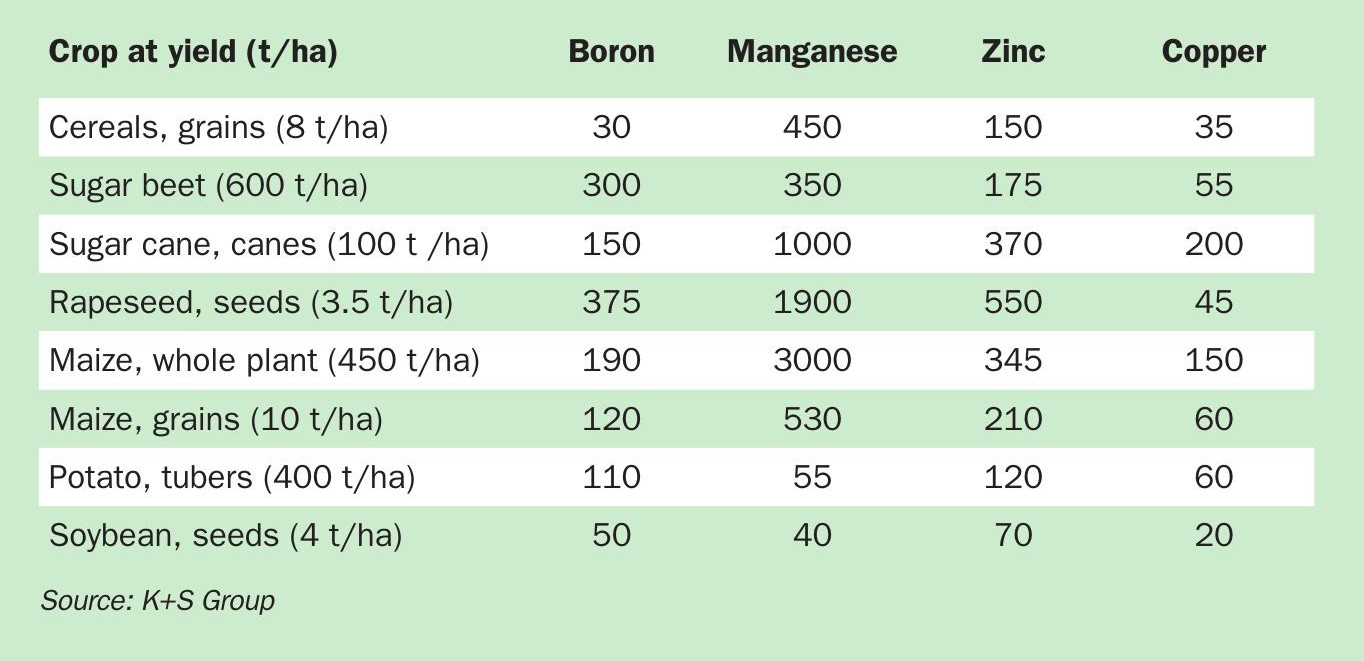
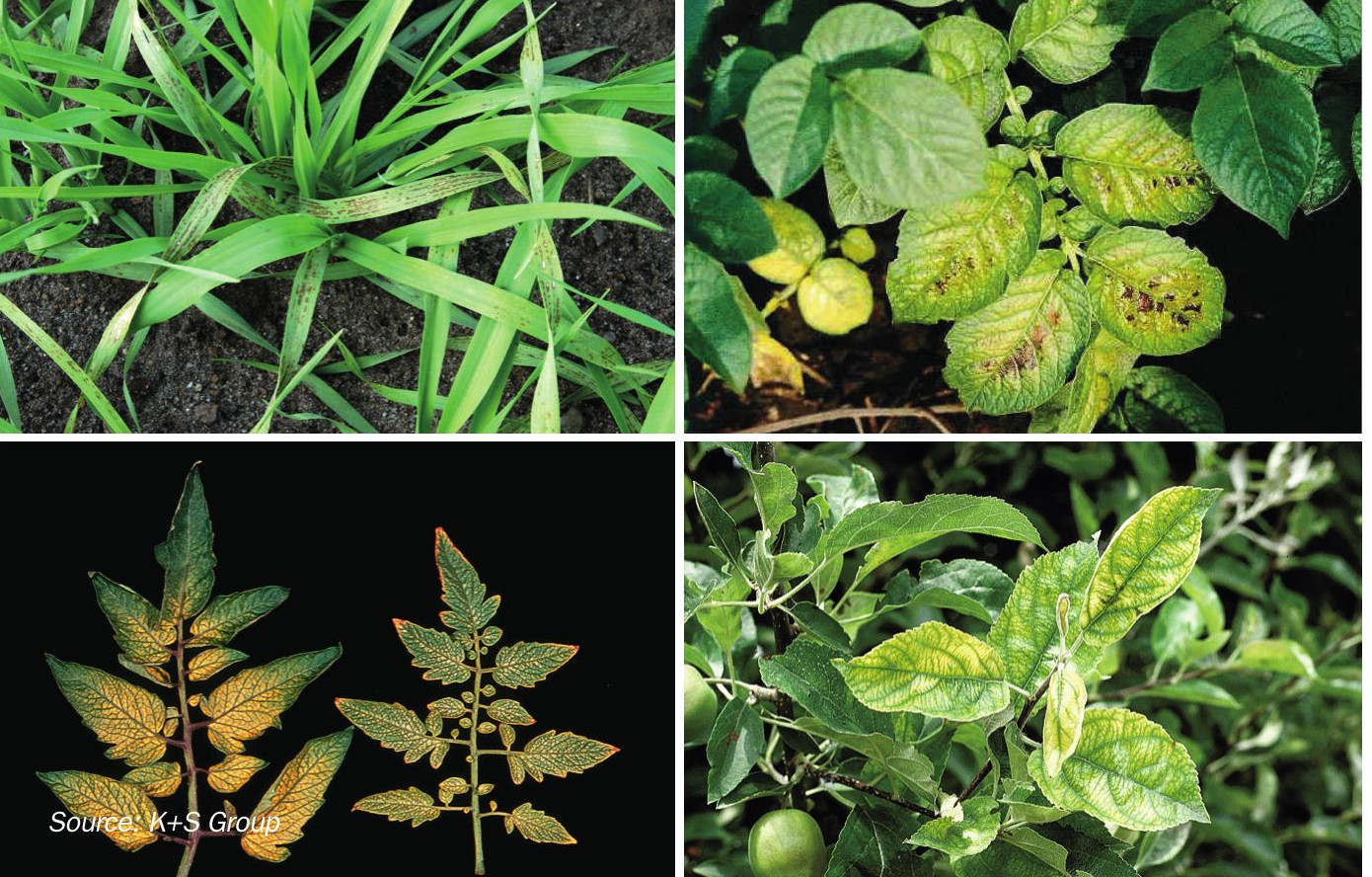
Manganese, enzymes, and photosynthesis
Manganese (Mn) has several vital roles within plants. When deficient, interveinal necrosis (Figure 1), leaf epinasty, inhibition in root lignification, and reactive oxygen species (ROS) formation can be observed. The main impacts of Mn deficiency in plants are summarised in Figure 2.
One of the main functions of Mn is to act as a metalloenzymes cofactor. Up to 101 Mn-containing enzymes have been identified in plants. Some 37 of these enzymes exclusively contain Mn, while the remaining can exchange Mn with other divalent metals6 . One noteworthy Mn-dependent enzyme is Mn-superoxide dismutase (Mn-SOD) located in the mitochondria and peroxisomes of plants. This catalyses the dismutation of superoxide radicals (O2 -) into oxygen (O2 ) and hydrogen peroxide (H2 O2 ) when water is present7 . Mn plays a major and valuable role by catalysing ROS (in this case, O2 -) as these are toxic and can cause cell death.
Zinc, oxidative stress, and membrane integrity
Zinc (Zn) has numerous roles within the biochemical and biological processes in plants. Growth is inhibited when plants are zinc deficient due to effects on specific hormones. Interveinal necrosis and chlorosis, and light-induced chlorosis and necrosis are also common consequences of Zn deficiency (Figure 3).
A zinc-containing enzyme (Cu/Zn-SOD) is involved in the scavenging of ROS. Consequently, higher concentrations of toxic ROS can accumulate in Zn-deficient plants exposed to high light intensity. Moreover, the activity of catalase (another ROS scavenging enzyme) is reduced when zinc is deficient, leading to the accumulation of damaging H2 O2 (Figure 4).
Zinc is also required for the structural and functional integrity of plant bio-membranes. Zinc protects lipids and proteins within membranes against oxidative damage from ROS, as it binds to the sulfhydryl and phospholipid groups of the membrane constituents, or forms tetrahedral complexes with cysteine residues from a polypeptide chain. The permeability of these membranes also increases due to higher rates of O2 – when Zn is deficient. This can lead to a leakage of low-molecular-weight solutes via roots8 . An optimal Zn supply is therefore required to lower membrane permeability and avoid these losses from cells.
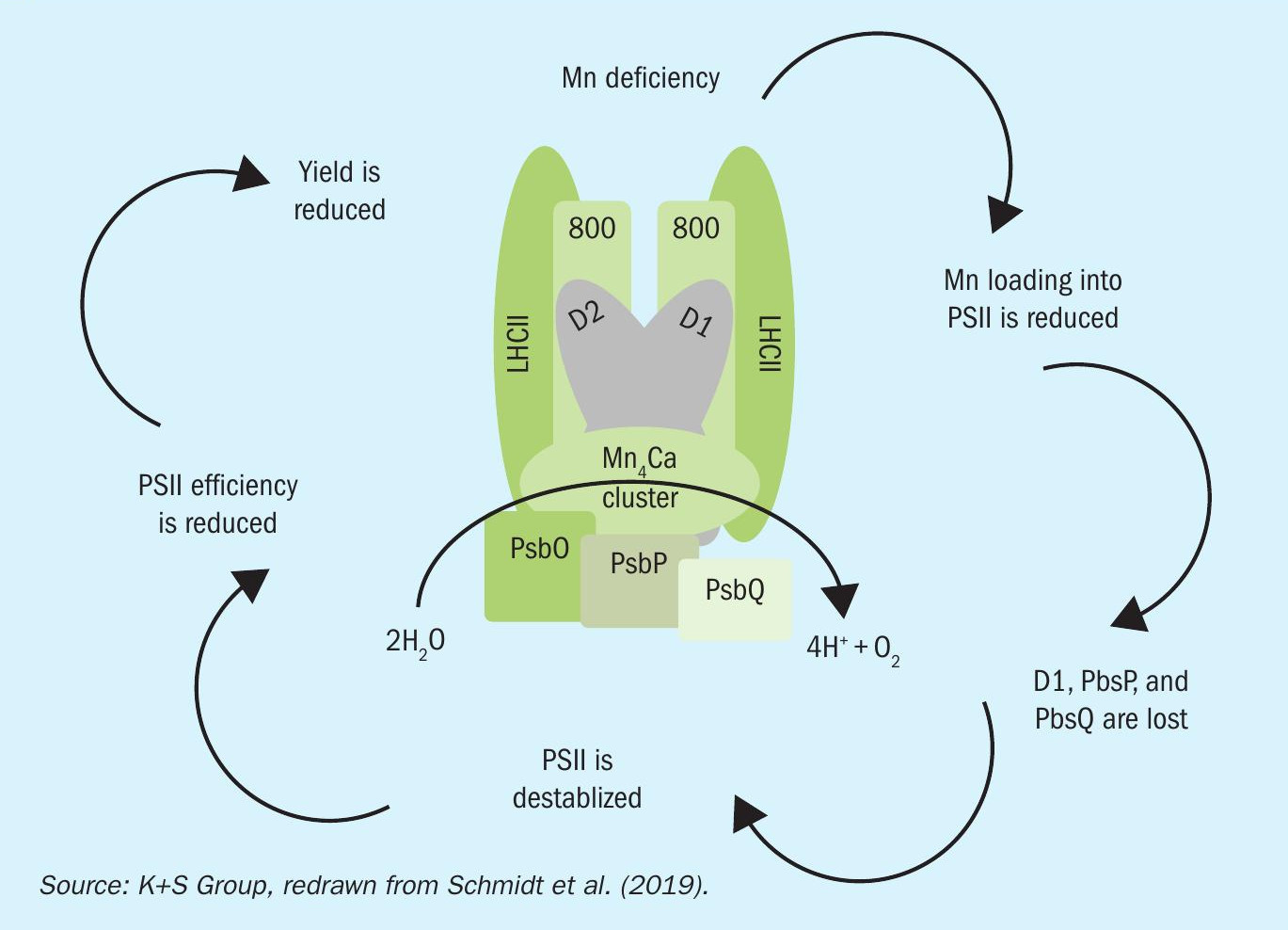
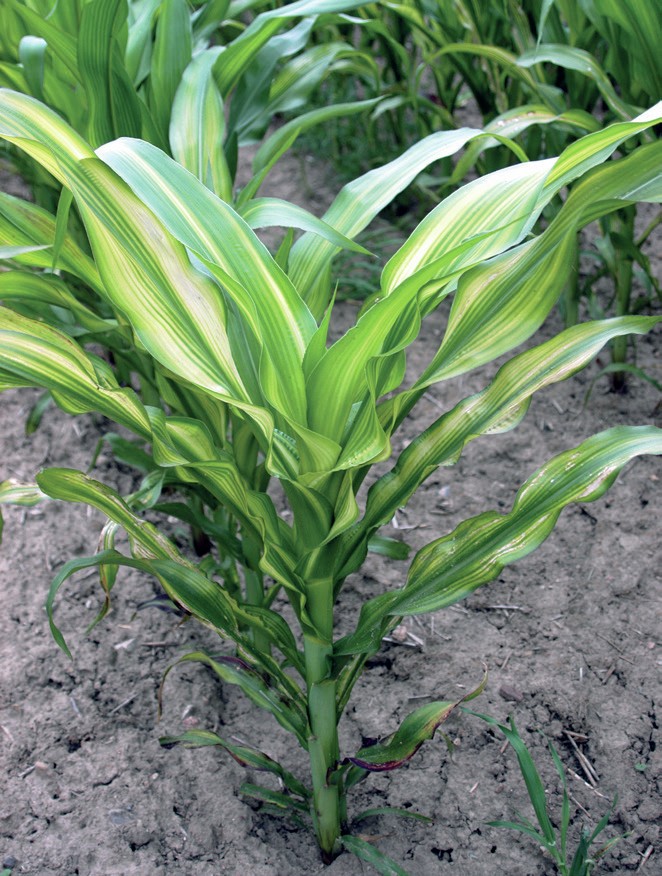
Boron – essential for cell wall structure and function
Boron (B) was first identified as an essential nutrient for plant growth in 1923 – yet its precise role in plant metabolism is still being investigated over a century later. While the role of boron in carbohydrate metabolism and sugar transport, in cell wall synthesis and structure, in lignification, and several other processes has been proposed, for example, only its role in cell walls has been demonstrated definitively. Interestingly, and unlike all the cationic micronutrients (Fe, Mn, Zn, Cu, Ni), boron is not an enzyme constituent.
Soil boron is available for plant uptake as boric acid. This weak acid (std pKa 9.25) is predominantly present as free acid [H3 BO3 or B(OH)3 ] in soils under neutral and acid conditions and as the borate anion [B(OH)4 -] in alkaline soils. Uptake is therefore strongly pH dependent. Boric acid, as an uncharged molecule, easily permeates root membranes in acidic conditions but its movement is restricted in alkaline soils. When boron concentrations in the soil solution are below sufficiency levels (<1 μM), plants activate specific transporters to regulate its uptake to meet their growth requirements.
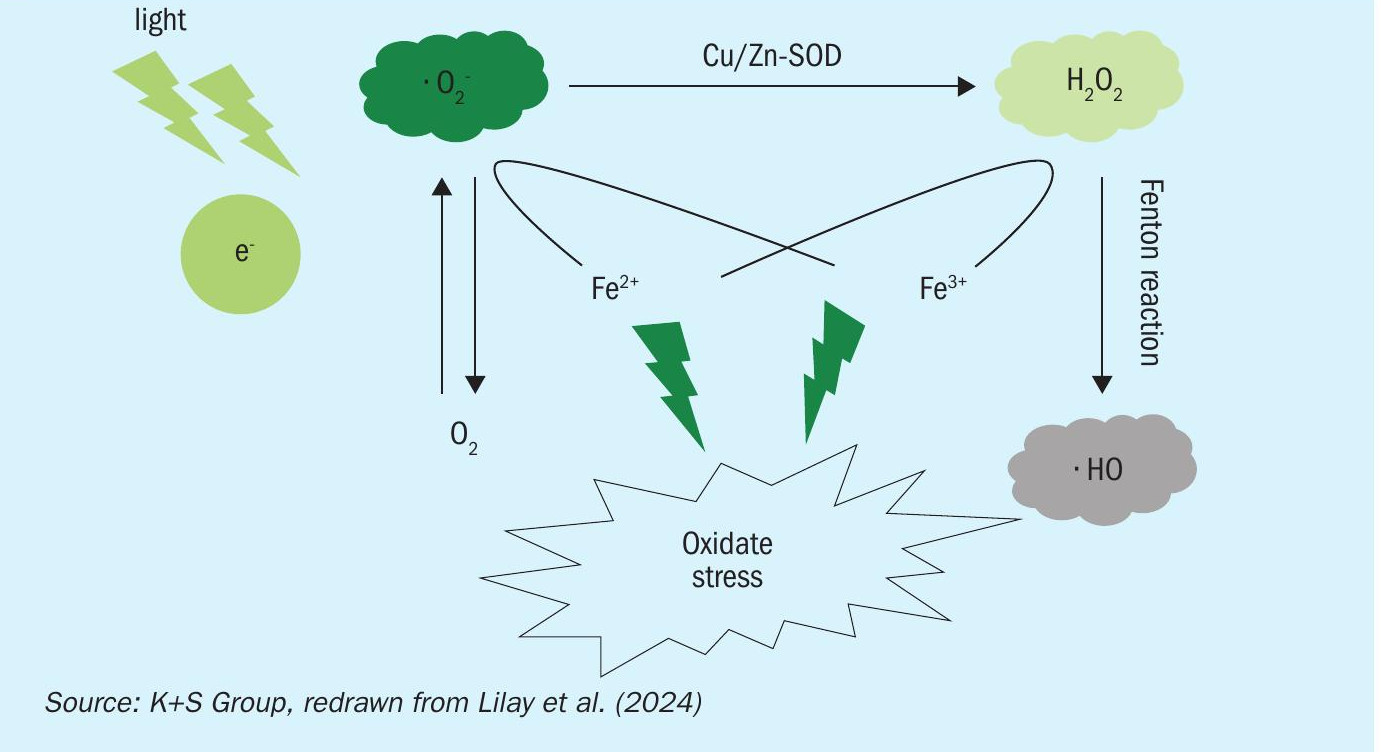
Boron transport within plants is a complex process that varies significantly between species, as it is influenced by the type of carbohydrates produced and transported within individual plants. In plants that generate and transport polyols like sorbitol or mannitol (e.g., many members of the rose family, including pome and stone fruits), boron can move freely in both the xylem and phloem, mirroring the behaviour of phloem-mobile elements such as potassium and magnesium.
In contrast, boron is not phloem-mobile in all the major field crops which use sucrose as their main carbohydrate. Movement is instead restricted to the xylem and driven by transpiration. Weakly transpiring plant parts and organs – like the shoot tip, buds, flowers, and fruits – are therefore prone to boron deficiency. Consequently, boron applied through the foliar fertilization of field crops, although immediately available for uptake, also stays where it is applied and does not translocate from mature leaves to the sites of new growth. Foliar applications of boron will therefore only have a limited effect in field crops unless repeatedly applied.
Boron’s role in plant nutrition – despite its importance – is still the least understood of all the nutrients. While its significance for cell wall integrity is well-established, boron does not appear to be involved directly in the synthesis of the cell wall itself.
The association of boron with pectins (heterogeneous polygalacturonates) through ester bonds is a key factor in its function within cell walls. The pectin matrix between the walls of adjacent cells is vital for structural tissue integrity. Its presence enhances the mechanical properties of the cell wall, such as stiffness and low porosity, which are essential for maintaining plant cell shape and vigour.
Monocots, like cereals and grasses, generally have a much lower boron requirement during exponential growth (typically 5-10 mg/kg DM) than dicotyledonous plants (typically 30 mg/kg DM), which include a wide variety of broad-leaved crops. This is because the cell walls of cereals contain much lower amounts of pectins (particularly rhamnogalacturonans II) that bind with boron and form stable structures.
Understanding these subtle intricacies and the different boron requirements of crops (Table 1) is vital for optimal plant nutrition and growth. The growth of pollen tubes is a particular developmental process that is highly sensitive to insufficient boron supply – this having serious consequences for pollination, fruit set, yield, and quality of all crops where generative organs are harvested.
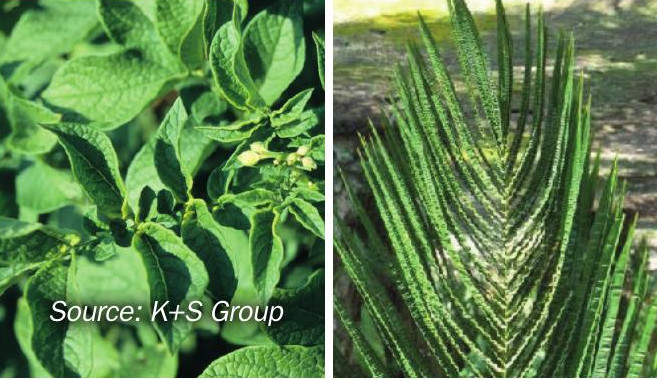
While its role in some metabolic processes is still being explored, boron deficiency is known to significantly disrupt the cell walls of plants (Figure 5), leading to a series of metabolic challenges. Boron deficiency can result in unusually thick and brittle cell walls, for example, that lack flexibility and strength. These defects can impede normal cell expansion, affecting tissue integrity and overall plant development. They typically interfere with the development of expanding tissues – like stems, bulbs and fruits – and results in cracks that provide entry points for pathogens and pests. Similarly, root elongation is also severely impaired by boron deficiency.
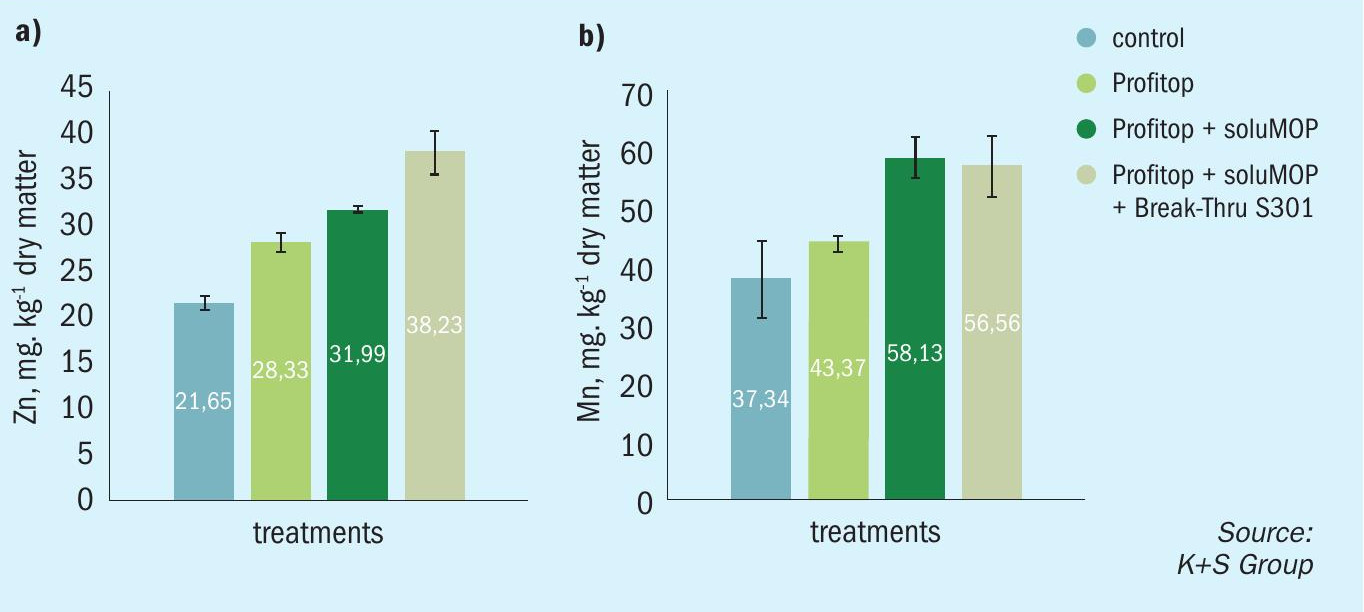
K+S trials and products
The following field trial results demonstrate the practical benefits of applying micronutrient enriched products to crops:
The micronutrient enriched fertilizer epsoPROFITOP® (12% MgO, 14% S, 5% Mn, 2% Zn and 1% Cu) was applied to an oat crop at 10 kg/ha. In an additional treatment, soluMOP® (60% K2 O) was applied at a rate of 1.5 kg/ha. Micronutrient uptake and translocation within the plants was then investigated by sampling and testing newly expanded leaves two weeks after these treatments. The results showed a pronounced increase in leaf zinc and manganese content (Figure 6).
Other field trials examined the effects of boron-containing fertilizers on the yield and boron status of oilseed rape (OSR) and sugar beet – two boron-loving crops (Table 1). As mentioned previously, the translocation of boron within arable crops after foliar application is limited unless applied several times.
The OSR trial was conducted on a loamy sand with optimal phosphorus status, very high magnesium status, and very low boron levels. All plots received:
- 196 kg/ha of nitrogen from urea, diammonium phosphate (DAP) and calcium ammonium nitrate (CAN)
- 45 kg/ha sulphur as ammonium sulphate nitrate (ASN)
- Potassium at a rate of 120 kg K2 O/ha apart from the control
- Boron as either foliar-applied epsoBOR-TOP® spray or soil-applied Korn-KALI® +B.
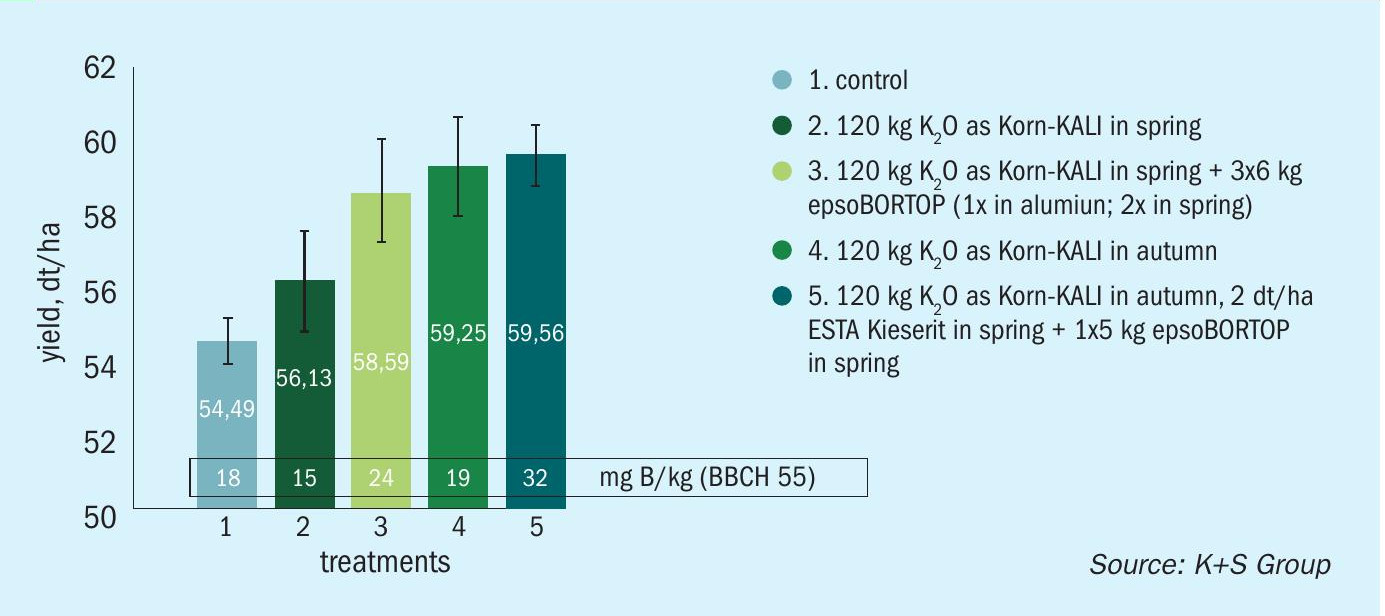
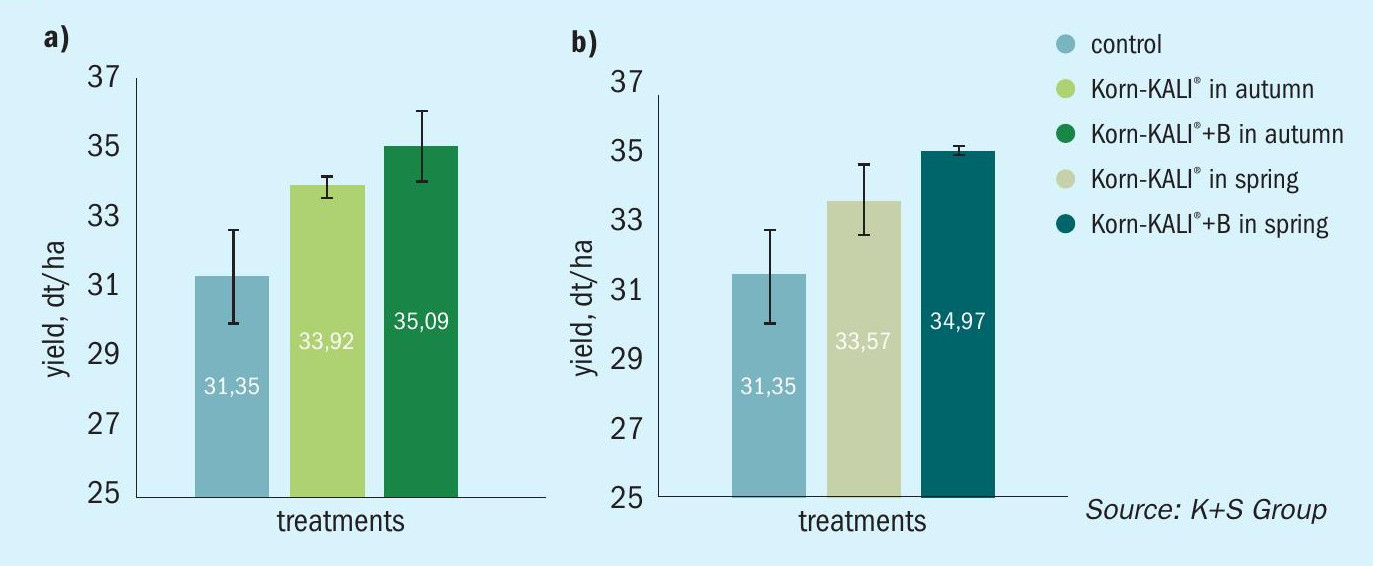
Results demonstrated that foliar fertilization using epsoBORTOP® effectively increased both OSR yield and the crop’s boron status, with soil-applied boron using Korn-KALI® +B resulting in an even better yield response. Combining both these foliar- and soil-applied approaches proved most effective (Figure 7).
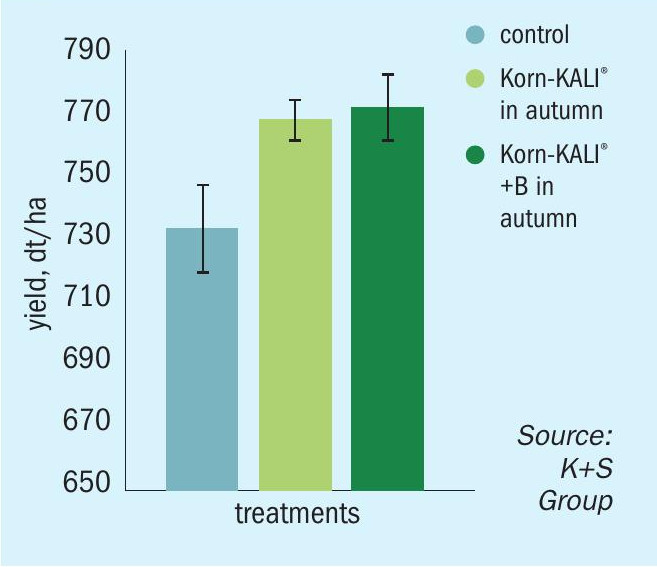
Positive yield responses were also observed in other trials on OSR and sugar beet, irrespective of whether boron was supplied in autumn or spring (Figures 8 and 9).
Micronutrient-enriched fertilizers from K+S Group
When crops have a deficiency, foliar fertilization can help provide the required nutrients to crops efficiently and rapidly. The epso family of products from K+S Group (Table 2) are water-soluble, highly efficient, and contain micronutrients at higher concentrations than typically seen in soil fertilizers.
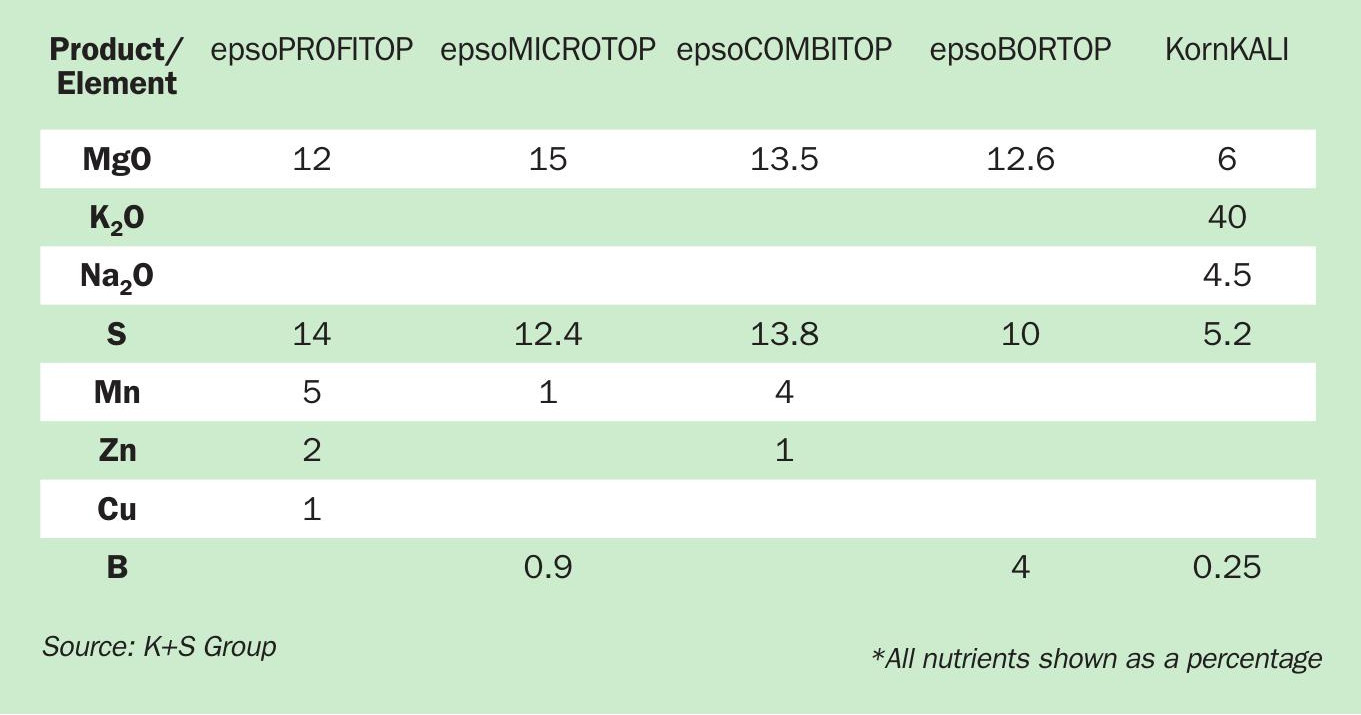
As well as micronutrients, epso fertilizers also contain magnesium and sulphur. Magnesium is vital for chlorophyll biosynthesis, photosynthesis, translocation of photoassimilates, and root growth. Sulphur, meanwhile, is necessary for nitrogen use efficiency and protein synthesis.
K+S Group offers the following four micronutrient foliar fertilizers:
- epsoPROFITOP® is suitable for crops such as potato, spring and winter cereals, and corn. 1-3 applications (5 kg/ha) at 2.5 percent concentration are recommended for optimum yields. Recommendations do, however, vary in different regions according to the nutrient content of the soil and crop yield expectations.
- epsoMICROTOP® dissolves well in water without leaving residues and can be easily mixed with plant protection products. Recommendations are: 3-4 application at a rate of 5-15 kg/ha for oilseed rape; 2-3 applications at 5-15 kg/ha for fruit crops; four application at 5-10 kg/ha for potato.
- epsoCOMBITOP® is suitable for various crops. Two applications of 10 kg/ha are recommended for optimum maize yields, whereas 2-3 applications of 5-10 kg/ha are recommended to efficiently deliver the nutrient requirements of cereals and vegetables.
- epsoBORTOP® is recommended for crops with medium to high boron demand. It can be mixed with plant protection products. Reducing the pH value of the spray tank is also welcomed. 2-3 applications at a rate of 5-7.5 kg/ha are recommended for optimal oilseed rape and sunflower growth. In sugar beet, 2-3 applications at 5-10 kg/ha are recommended.
Additionally, K+S offers Korn-KALI® +B as a multi-nutrient, granular fertilizer containing K, Mg, S, Na and B. Its application, which is usually governed by the potassium requirement of the crop, also supplies a range of the other valuable nutrients in water-soluble, plant-available form. It is suitable for many chloride-tolerant crops. Korn-KALI® +B does not affect soil pH and its efficacy is not pH-dependent either.
References