Sulphur 417 Mar-Apr 2025
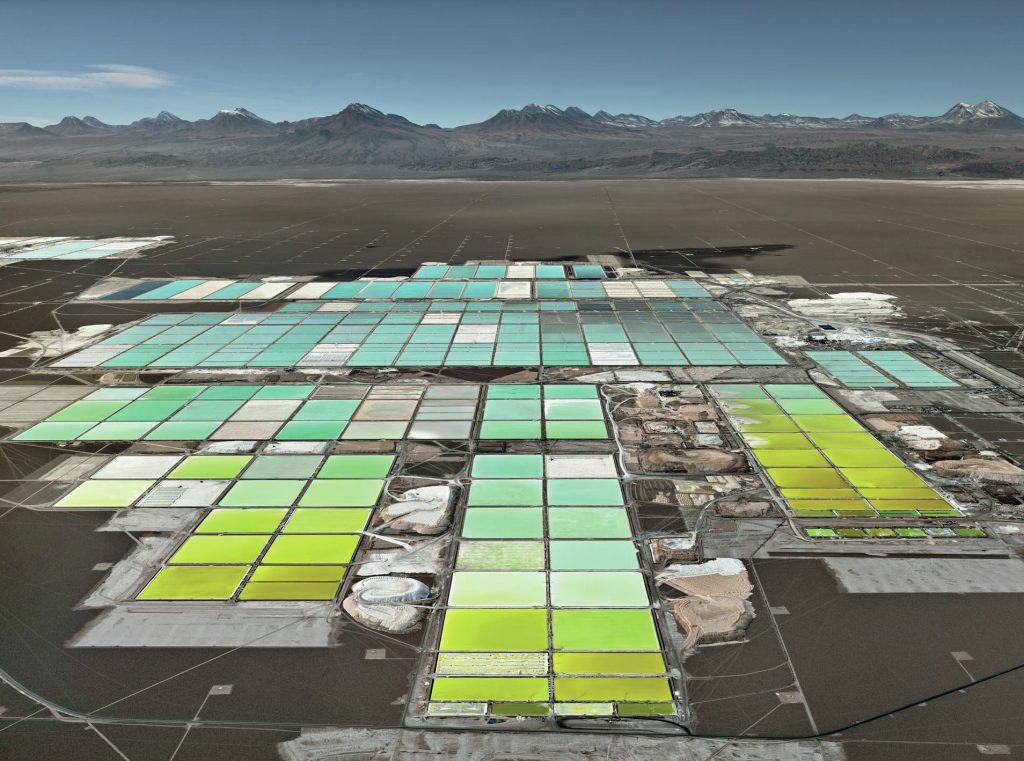
19 March 2025
Lithium production and acid demand
LITHIUM
Lithium production and acid demand
Rapidly increasing lithium production is projected to require several million t/a of sulphuric acid in the next few years, with China, the USA and Australia the main consumers.
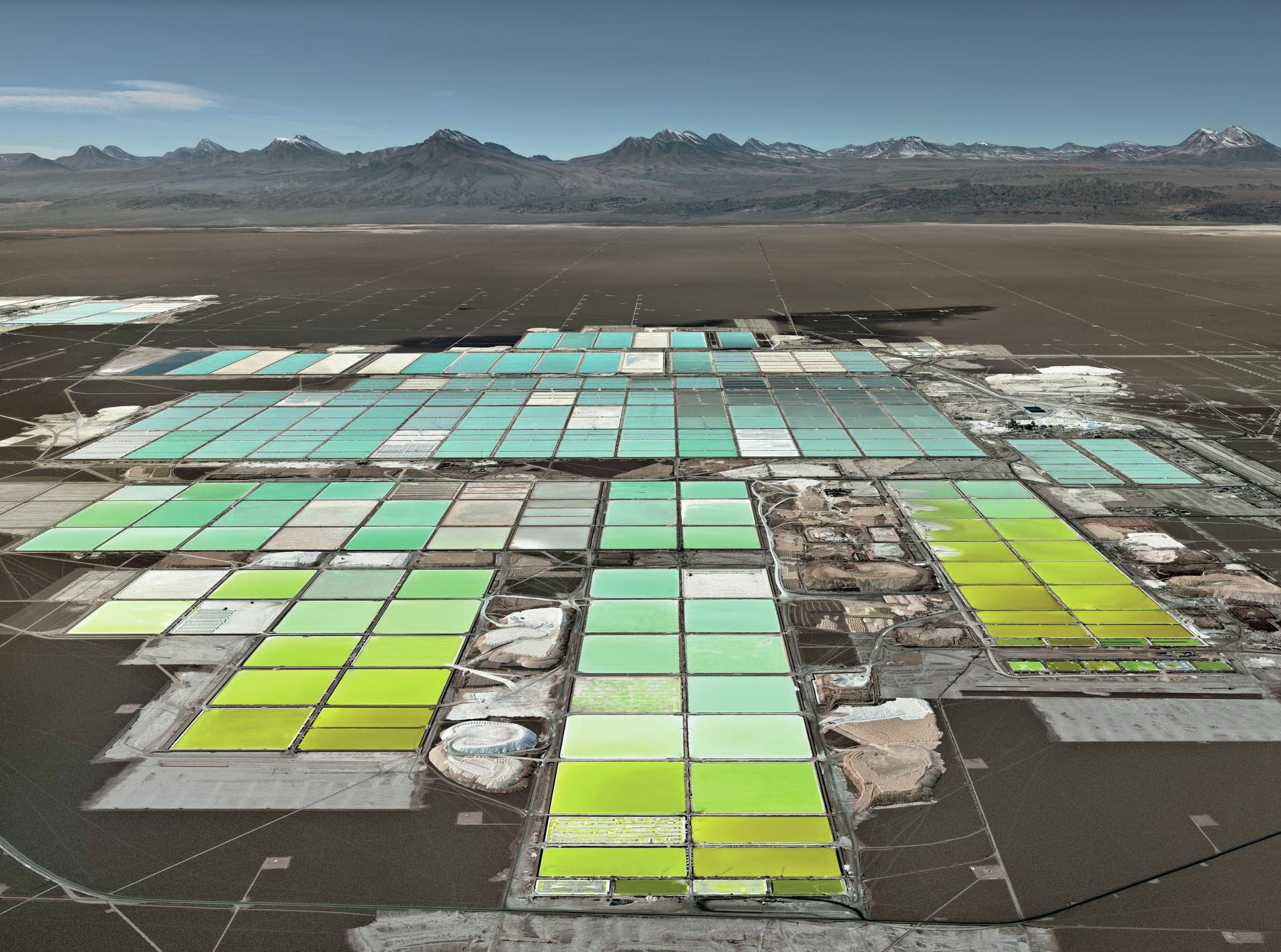
Global demand for lithium is increasing rapidly, driven mainly by its use in various battery technologies. As Figure 1 shows, demand has tripled in the past five years, and is projected to double in the next five.
Lithium production
Lithium deposits are not widely spread globally. The US Geological Survey estimates that, of around 30 million tonnes of economically attractive lithium reserves, 9.3 million tonnes are in Chile and 7.0 million tonnes in Australia, 4 million tonnes in Argentina, 3 million tonnes in China and 2.8 million tonnes in the USA. These five countries collectively represent 90% of all deposits.
There are basically three main methods of producing lithium; from brines, either by solar evaporation or a new process called direct lithium extraction (DLE), or conventional hard rock mining of lithium containing ores, mainly spodumene, a lithium aluminium silicate. Rock mining was the dominant method of extraction until the 1990s, when recovery from brine deposits became the most widely used source. Surface or underground salt deposits and brine pools are mixed with water and pumped into large evaporation pools to concentrate the lithium, using calcium hydroxide to precipitate out unwanted metals like magnesium and boron, before being processed using soda ash (sodium carbonate) to produce lithium carbonate. However, brine processing is a slow process and very water intensive; a resource not always widely available in the salt flat regions where the processing takes place. As a result, the pendulum has swung back towards rock mining in recent years. Rock mining is followed by conventional mineral processing: crushing, concentration, and roasting and/or leaching using sulphuric acid, to obtain a lithium concentrate. As in brine-based lithium extraction, lime is added for the removal of magnesium (a constituent element in spodumene), and soda ash is used to precipitate lithium carbonate from the final purified, filtered solution. In Nevada, lithium exists in clays which are treated with sulphuric acid to extract the lithium.
Direct lithium extraction (DLE) has been used in China and South America. Brine is extracted from the basin aquifer and pumped to a processing unit where a resin or adsorption material is used to extract lithium, while spent brine is reinjected into the basin aquifers. The resin adsorbs lithium chloride, which is then stripped using water. The lithium solution is then further concentrated via reverse osmosis and mechanical evaporation stages before standard industry processes are used to produce battery-grade lithium.
In 2023, rock mining accounted for 66% of all lithium production, mainly in Australia, solar brine evaporation another 24%, with South America and China the main locations, and DLE about 10%, again in South America and China. Figure 2 shows the main lithium producing nations at present.
Lithium demand
Rapidly increasing demand for mobile or remote electrical power, especially from the vehicle sector, is driving demand for lithium. High power density per unit weight and rechargeability are leading them to replace older battery technologies like alkaline batteries for portable electronics or lead acid cells for vehicles. There are roughly speaking six main lithium battery technologies; LFP has become a popular choice due to cost, particularly in China, and particularly among Chinese smart EV producers, such as NIO, Xiaomi Auto and Li Auto. The world’s largest EV manufacturer, BYD, recently announced that they have now equipped more than 2 million new energy vehicles with LFP starter batteries, instead of the incumbent lead-acid – reportedly reducing lead consumption by 20,000 t/a. LFP batteries are lighter, and while initially costlier than lead-acid, may last up to ten times longer – meaning lower costs over the battery lifespan.
• Lithium iron phosphate (LFP) batteries use phosphate as the cathode material and a graphitic carbon electrode as the anode. LFP batteries have a long life cycle with good thermal stability and electrochemical performance. Low cost means that LFP has been a popular choice in China for EV batteries in spite of lower energy density than some other technologies.
• Lithium cobalt oxide (LCO) batteries have high specific energy but low specific power. This means that they do not perform well in high-load applications, but they can deliver power over a long period. LCO batteries were common in small portable electronics such as mobile phones, tablets, laptops, and cameras. However, they are losing popularity to other types of lithium batteries due to the high cost of cobalt and concerns around safety.
• Lithium manganese oxide (LMO) batteries use lithium manganese oxide as the cathode material. This chemistry creates a three-dimensional structure that improves ion flow, lowers internal resistance, and increases current handling while improving thermal stability and safety.
• Lithium nickel manganese cobalt oxide (NMC) batteries combine the benefits of the three main elements used in the cathode: nickel, manganese, and cobalt. Nickel on its own has high specific energy but is not stable. Manganese is exceptionally stable but has a low specific energy. Combining them yields a stable chemistry with a high specific energy. NMC batteries are used in some electric vehicles.
• Lithium nickel cobalt aluminium oxide (NCA) batteries offer high specific energy with decent specific power and a long lifecycle. This means they can deliver a relatively high amount of current for extended periods. They are also a popular choice for electric vehicles, such as Teslas.
• Lithium titanate (LTO) batteries replace the graphite in the anode with lithium titanate and use LMO or NMC as the cathode chemistry. Wide operating temperature range and long lifespan make them suitable for aerospace applications and stationary power.
Chinese investment in LFP production has led to overcapacity and a fall in lithium prices; in 2024 lithium carbonate prices averaged nearly two-thirds lower than in 2023. However, CRU anticipates that demand will catch up with supply during 2025, in part due to some curtailments in lithium supply in e.g. Australia, with production down 14% on forecasts. LFP cathode production is also at record levels, growing 94% in 2024. Soaring LFP output, powered by strong Chinese EV sales and booming energy storage demand, will be a key driver of the lithium price recovery in 2025 in spite of slower growth in EV purchases outside pf China.
In addition, there has been booming demand from the energy storage sector. Since 2022, battery energy storage systems (BESS) demand has grown sevenfold, far outpacing e-transportation. Much of this growth is being driven by the economics of power storage, new supportive tender mechanisms, longer term contracts, arbitrage and grid stabilisation services – with a third of last years’ installations in major markets not directly connected to renewable energy installations. In addition, BESS demand will benefit from solid growth in the solar PV sector, with solar module costs and prices expected to remain low in 2025. BESS usage has also benefitted from LFP overproduction, with storage system bid prices falling below the $100/kWh level in China. Although LFP alternatives like sodium-ion will start to proliferate, cost parity is not likely to be achieved until later in the decade. Overall, CRU expects BESS demand to grow more than 70% year on year in 2025 for the third consecutive year.
As shown in Figure 1, the prospects for lithium continue to be bright over the medium term, with some analysts predicting a shortage from 2030 onwards due to lack of investment in new mining capacity.
Recent industry developments
Russian nickel-copper producer Norilsk Nickel (Nornickel) has agreed to send copper concentrate and by-product sulphuric acid to China for processing and, in return, will receive technologies from Chinese partners to enable production of battery materials from Russia’s lithium deposits, as part of a new, four-year strategy for moderate growth. Nornickel is working with Russian nuclear power supplier Rosatom on a lithium project in the Murmansk region. The plan is to start construction of an open-pit mine at Kolmozerskoye in 2026 with completion in 2029 and production beginning the following year.
China’s Zijin Mining Group has committed to spending $1-3-1.6 billion to construct a 100,000 t/a zinc smelter with capacity for 200,000 t/a of sulphuric acid as a first phase, with a lithium carbonate extraction plant capable of producing 60,000 t/a of battery-grade lithium carbonate to follow in a second phase.
BHP suspended its Nickel West operations from October 2024 until at least February 2027. The decision will remove acid supply from the company’s Kalgoorlie nickel smelter, which supplied 550,000 t/a to the regional acid market. While BHP used 250,000 t/ for ammonium sulphate production, external demand is split between 100,000 t/a of lithium-based acid consumption around Kwinana, as well as rare earths and other uses, who will need to import acid from outside the region at increased cost. Nevertheless, the lithium operations remain viable with or without the Kalgoorlie supply. Lithium-based acid demand is forecast to increase from 90,000 t/a in 2023 to over 400,000 t/a by 2028 due to mining expansions. Due to the scale of growth, an increase in imports will be required. Kalgoorlie’s closure may therefore necessitate that imports increase sooner.
US trade policy
The incoming Trump administration has taken a very different tack on the environment from its predecessor and this may impact upon the EV market. It seems increasingly likely that president Trump will scrap one or both of the 30D and 45X IRA tax credits. The Clean Vehicle Credit (30D) is believed to be at higher risk of repeal compared to the Advanced Manufacturing Production Credit (45X), as the latter attracts significant foreign investment and creates jobs primarily in Republican states. Although Germany’s late-2023 removal of consumer subsidies prompted a significant decline in BEV sales, a similar US move is unlikely to be as impactful under the IRA’s current guise, because Foreign Entity of Concern rules around critical minerals are so stringent that barely any EVs will qualify from January 2025.
The Trump administration may also consider relaxing emissions standards, which could incentivise OEMs to shift back towards more profitable internal combustion models. Rollbacks of European emissions standards, or the US’ CAFE and EPA rules would be a major blow, although probably over longer timescales given that OEMs operate across multi-year development cycles.
Meanwhile, the escalating Sino-American trade war has seen the US place tariffs on a range of Chinese materials – while China is reportedly considering tightening export controls on various battery-related technologies. The US relies on Chinese LFP battery imports to support its booming energy storage system market. BESS operators have very limited alternatives, at least for the next few years. The US also lacks domestic cathode and graphite anode production, relying on imports from China and South Korea. Higher tariffs will inflate battery and EV costs, ultimately burdening consumers. Around 20% of US EV sales are imported from the EU and Mexico, making up over half of total EV imports, and despite growth in the domestic battery sector, supported by IRA 45X subsidies, 31% of US consumption still relies on imports, mainly from China. It seems likely that the current trade war may damage the uptake of EVs in the US, though at present the market is being led by growth in China.
Lithium production expansions
As Table 1 indicates, the main expansions in lithium capacity and concomitant acid demand over the next four years are likely to be in the US, China and Australia. The start of the Lithium Americas and Ioneer projects in the United States will add 1.4 million t/a of acid consumption from 2028 and 1.9 million t/a in 2029. While the US projects are relatively high cost lithium producers and have depended on Department of Energy funding for getting off the ground, Trump’s freeze on DoE funding is unlikely to affect projects which have already agreed funding.
Additional Chinese production will represent another 1.25 million t/a of acid demand, and Australia another 400,000 t/a, at a variety of sites mainly across Western Australia, where there has been a focus on cost reduction of lithium production. A number of African projects are expected towards the end of the forecast period but construction has yet to begin and no supply is likely before 2030.
Overall, lithium production is likely to be consuming an extra 4 million t/a of acid by 2029, but as battery use continues to grow into the 2030s, this figure is likely to continue to increase rapidly in the early years of the next decade.