Sulphur 391 Nov-Dec 2020
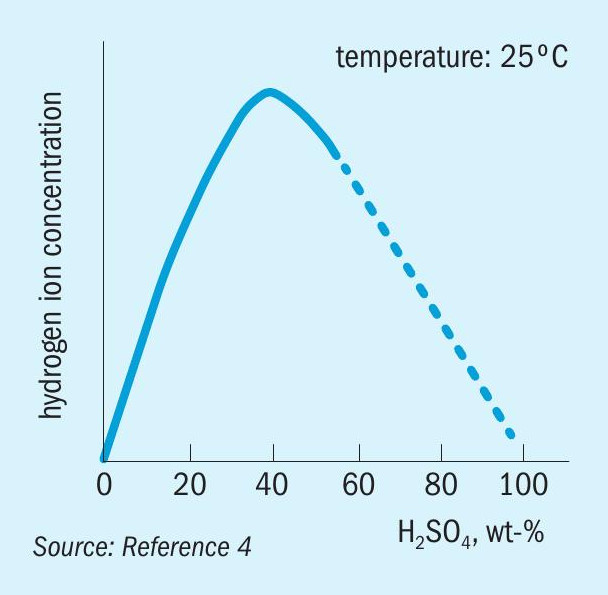
30 November 2020
Materials for pumps, valves, and piping in sulphuric acid service
CORROSION RESISTANT MATERIALS
Materials for pumps, valves, and piping in sulphuric acid service
Several highly corrosion resistant materials are available today for use in handling process fluids encountered in the production of concentrated sulphuric acid. These alloys, properly selected for the operating conditions, provide the benefits of long operating life under harsh operating conditions extending the period of uninterrupted production cycles and lowering the incidence of catastrophic equipment failure. In this article, M. J. Cooke of Weir Minerals discusses materials of construction for pumps, valves and piping used in the production of sulphuric acid.
Pumps, valves, and piping used in the production of concentrated sulphuric acid are subjected to a media that is hot, corrosive, and often abrasive. In order to maintain performance throughout a reasonable service life, the designers of such equipment must select materials that are suitable for these fluid properties, especially for those critical components subject to high fluid velocities and turbulence.
Design considerations
Pumps, valves and piping, respectively, pressurise, control and direct process fluids. In addition to being exposed to the corrosive effects of the fluid, this equipment is subjected to high velocities (to 30 m sec in the case of pump components) and turbulent flow behaviour that can cause wear by erosion. Further complicating the issue is the presence of entrained solids that add the potential for abrasive wear.
Pump designs require that close dimensional tolerances be maintained in order to insure maximum hydraulic efficiency and performance, particularly between impeller/casing rings, and sleeve bearing components. Valves require the same maintenance of dimensional tolerances between discs/plugs and their seating surfaces, in order to provide precise control and/or tight shut-off according to their function. The perfect material of construction for these critical components is therefore one that resists corrosion over a usable range of acid concentration and operating temperatures, is hard enough to offer abrasion resistance when required, and has non-galling characteristics.
The selection of materials for the principal “body” components of pumps and valves (i.e. volutes and valve bodies) and piping must consider not only the potential effects of abrasion and corrosion but must have enough mechanical strength to contain the fluid pressure over a reasonable lifetime. Therefore, to build a useful and cost-effective product, the design and choice of materials must be optimised according to the nature of the fluid and the required conditions of service.
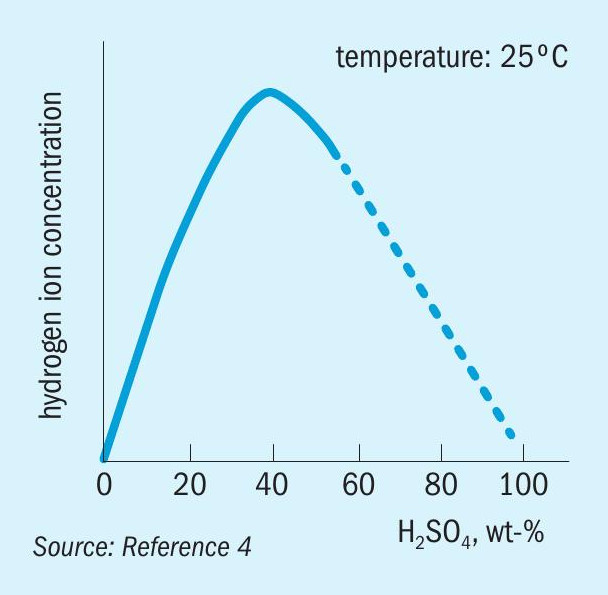
Cost effective pumps, valves and piping can be designed and supplied to meet flow sheet requirements in sulphuric acid plants and will yield good service life provided they are operated within the specified ranges. However, even the best-designed equipment will fail if process conditions are violated. The four primary causes of (unexpected) corrosion are: acid leaks, acid strength out of range, acid temperature too high, and acid flows that are too high.
Corrosion in sulphuric acid
Modern sulphuric acid plants handle acid strengths normally in the range of 93% to 99.5% and, in some plants various concentrations of oleum. The corrosion resistance of alloys within this relatively small concentration range can vary widely and can be difficult to predict.
The corrosiveness of sulphuric acid depends upon many factors, particularly temperature and concentration. However, whereas increases in temperature generally give a corresponding increase in corrosion rate, increasing the acid concentration can increase or decrease the corrosion rate depending upon the overall conditions. This effect is attributed to the fact that the hydrogen ion concentration in sulphuric acid increases with concentration, but at higher levels decreases again, as shown in Fig. 1. The hydrogen ion concentration directly effects the rate of the cathodic reaction and thereby the rate of anodic dissolution.
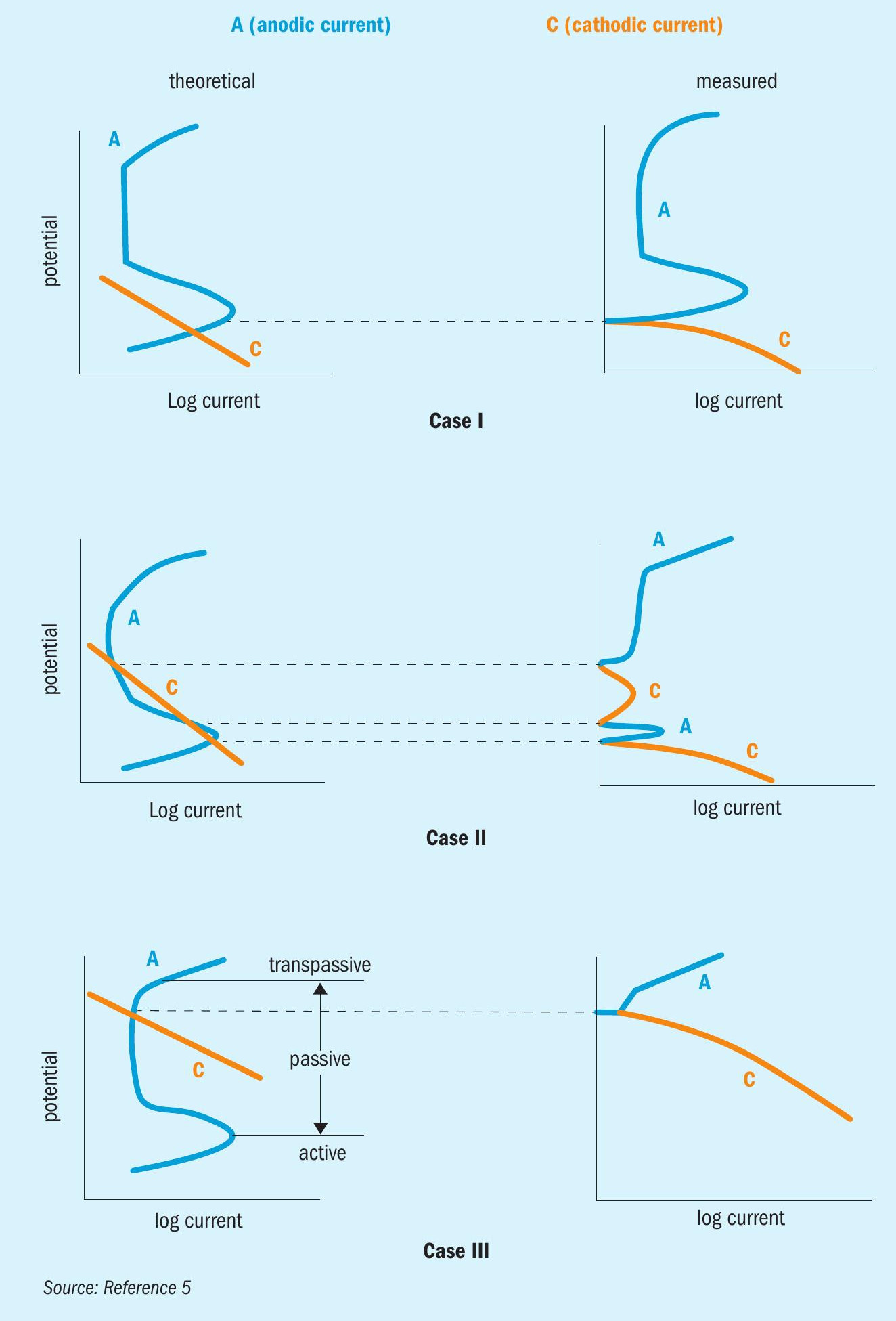
Additionally, corrosion rates can also be critically affected by other variables. The presence of oxidising or reducing impurities, the presence of chlorides, velocity effects, and galvanic effects may alter the serviceability of a particular material of construction. These influences can make predicting actual corrosion rates for a given material problematic.
The corrosion of metals in sulphuric acid is complex. However, the electrochemical behaviour of most metal alloys falls into three categories: active, passive, or active/passive (Fig. 2). For active behaviour (Case I), the corrosion potential is in the active region and a wide range of corrosion rates is possible. For passive behaviour (Case III), the corrosion potential is in the passive region; these alloys generally passivate spontaneously and exhibit low corrosion rates. For active-passive behaviour (Case II), both active and passive behaviour is possible. This can lead to the possibility of unpredictable and erratic corrosion rates, depending on the environment.
Cast irons
Cast iron has been used for many years in the construction of pumps, piping and other components in the manufacture of concentrated sulphuric acid. Today, gray iron, ductile iron, and several specialty irons continue to be used with good success in this service.
Like carbon steel, which is used in handling concentrated sulphuric acid at ambient temperatures under static and low velocity conditions, cast iron derives its corrosion resistance primarily from the formation of an iron-sulphate film. This sulphate film is highly protective, unless physically disturbed. The actual corrosion rate depends on the temperature, acid concentration, iron content, and flow, because these parameters determine the rate of dissolution of the protective sulphate film. However, compared to carbon steel, the higher carbon and silicon content of cast irons leads to conditions favouring superior resistance at higher velocities and elevated temperatures. Gray cast iron normally should not be used in the oleum range because of a tendency toward catastrophic cracking caused by a reaction between the free SO3 and the graphite flakes in the iron.
Lewis’ L-14® iron, introduced in 1980, is used extensively in larger acid pumps and gate valve products for principal body components where high dimensional requirements are not of paramount concern for hydraulic performance (volutes, elbows, discharge pipes, shaft columns and valve bodies). Alloying elements control the microstructure of the iron matrix in such a way as to provide favourable graphite dispersion. This iron resists “oleum cracking” and used in heavy-walled sections providing good service life in concentrated acid to temperatures approaching 135°C (see Fig. 3).
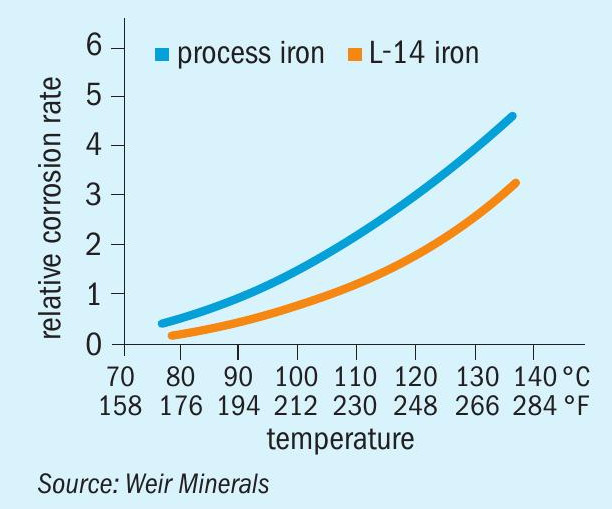
Several specialty ductile irons have been used extensively in piping around acid towers. Furnished in very heavy-walled sections, they have good ductility, and form a tough sulphate film that resists corrosion. Sizing the piping so that fluid velocities do not exceed 1-2 m/sec generally precludes erosive wear in acid piping.
Stainless steels and nickel based alloys
The corrosion resistance of stainless steels and most nickel-based alloys is complex due to the active-passive nature of the alloys. Resistance to corrosion depends upon achieving electrochemical passivity – Case III behaviour. Passivity is achieved by the surface formation of tightly adhering, crack-free, complex oxide films that protect the underlying metal. The formation and maintenance of these oxide films require oxidising conditions. Therefore, highly aerated solutions are much more suitable than air free ones. Similarly, the presence of oxidising impurities stabilises the passive film, markedly improving the corrosion resistance. Essentially, chromium and silicon are the two elements that promote the formation and adherence of these films. In addition, corrosion resistance can be often enhanced in mildly oxidising environments, and those containing reducing impurities, by the addition of nickel, molybdenum, and copper to the alloy. Other alloying elements are then added in order to adjust the metallurgical and mechanical properties of the final alloy.
Generally, for austenitic stainless steels (304, 316 types), stable passivity is achieved at ambient temperatures in the very low and very high concentrations and in oleum. Higher austenitic stainless steels (the 20-type alloys) are usually the first considered when the sulphuric acid environment is too corrosive for 300-series stainless steels or cast iron (see isocorrosion diagram, Fig. 4). The nickel-base alloys have superior resistance up to 95% concentration because of their high alloy content. Frequently, low corrosion rates occur in both the active and the passive corrosion states. Thus, reliable corrosion behaviour is achieved over a wide range of concentrations, temperatures, and impurity levels.
The use of highly corrosion-resistant nickel-based alloys used in the critical components of Lewis sulphuric acid pumps dates back to 1925, about the time these alloys became commercially available. The nickel base permitted higher additions of the desired alloying elements as compared to iron-based alloys. The early alloy, Ni-22Cr-7Mo-6.5Cu exhibited a corrosion rate of less than 0.25 mm/y in 98% acid to 90°C with operating velocities to 30 m sec. However, this alloy, which was fully austenitic, could not be hardened and was only available in cast form.
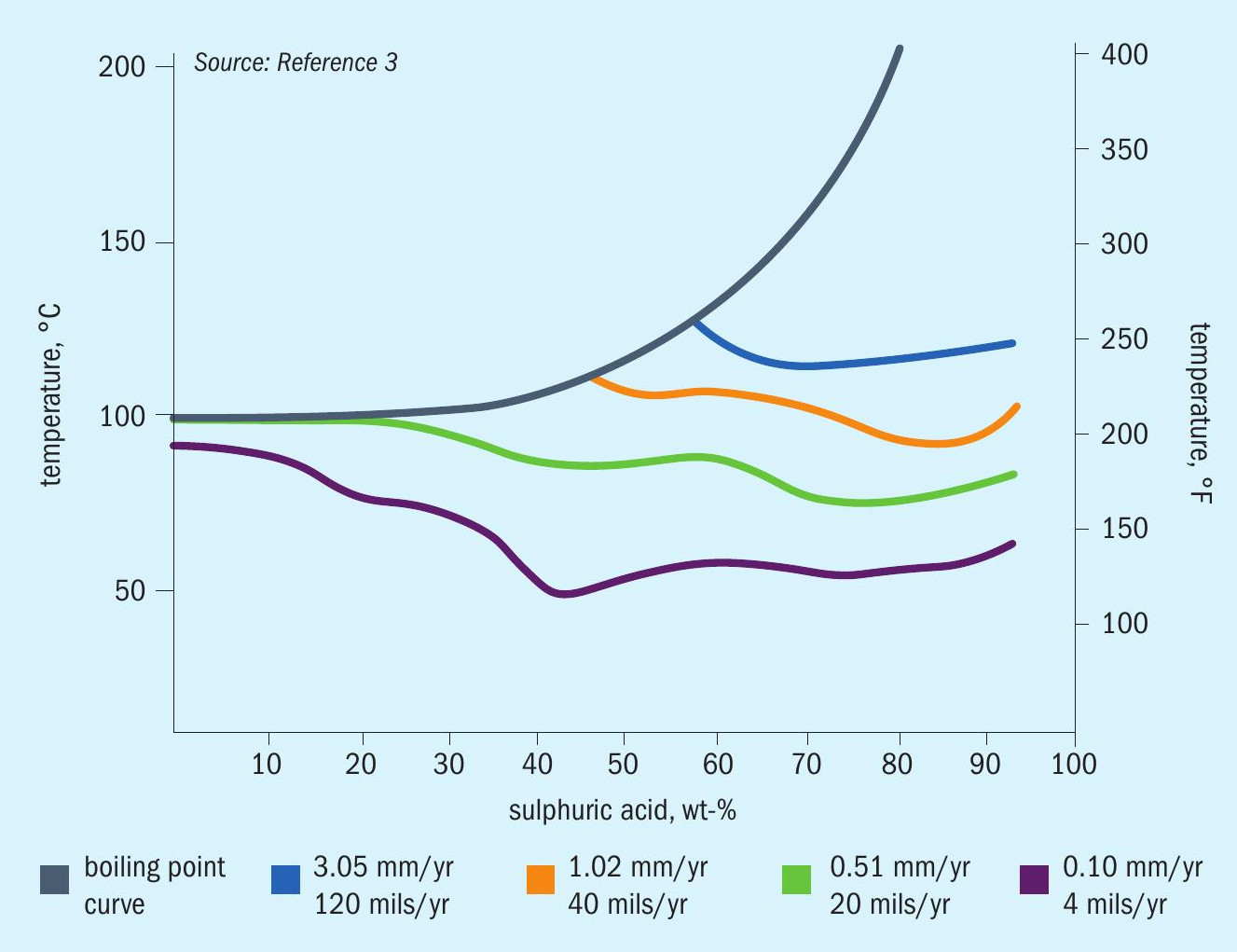
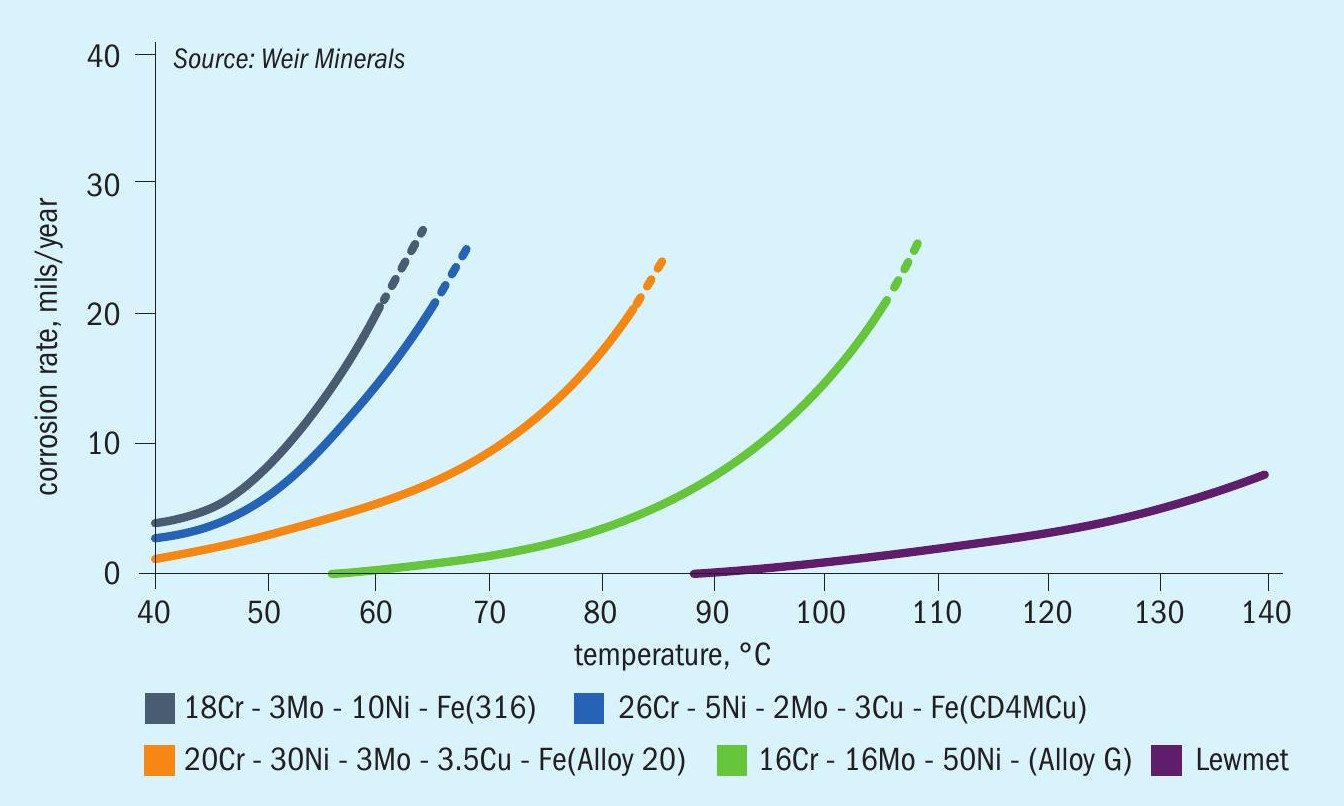
In 1940 Alloy 20, Fe-20Cr-30Ni-3Mo3.5Cu was introduced. While offering utility in 98% acid to 90°C operating temperature, the resistance to corrosion-erosion declines rapidly above this temperature. Alloy 20 is a fully austenitic alloy available in both cast and wrought form and cannot be hardened.
Lewmet® 33/44, Ni-31Cr-3Si-3Mo-3Cu, was introduced by Lewis Pump in the early 1970s. The corrosion rate of this alloy is presented in Fig. 5. This alloy also exhibits excellent corrosion resistance in 93-100% sulphuric acid and all concentrations of oleum. There is virtually no increase in the corrosion rate due to erosive wear at velocities to 30 m/sec. This alloy is hardenable to 400 BHN (45Rc) to provide excellent galling and abrasion resistance with no detectable loss in the corrosion rate. These characteristics make Lewmet® the ideal material for use in manufacturing close clearance critical components for pump sleeve bearings and impeller/casing rings and for valve discs/plugs and seats.
Subsequent to the introduction of Lewmet® 33/44, a wrought version of this alloy was developed. Introduced as Lewmet® 66, this modification has the same corrosion resistance as its parent alloy, is available in both cast and wrought forms, but cannot be hardened.
High silicon stainless steel alloys were developed in the mid-1980s. Nominally Fe-18Cr-18Ni-5Si, these alloys possess outstanding corrosion resistance in 98% sulphuric acid to 140® C. However, the corrosion rates can increase dramatically with decreasing acid concentrations and with acid concentrations in excess of 99.5% through the oleum range. Corrosion protection is obtained by the formation of a tenacious silicon-rich film formed on the surface during initial exposure.
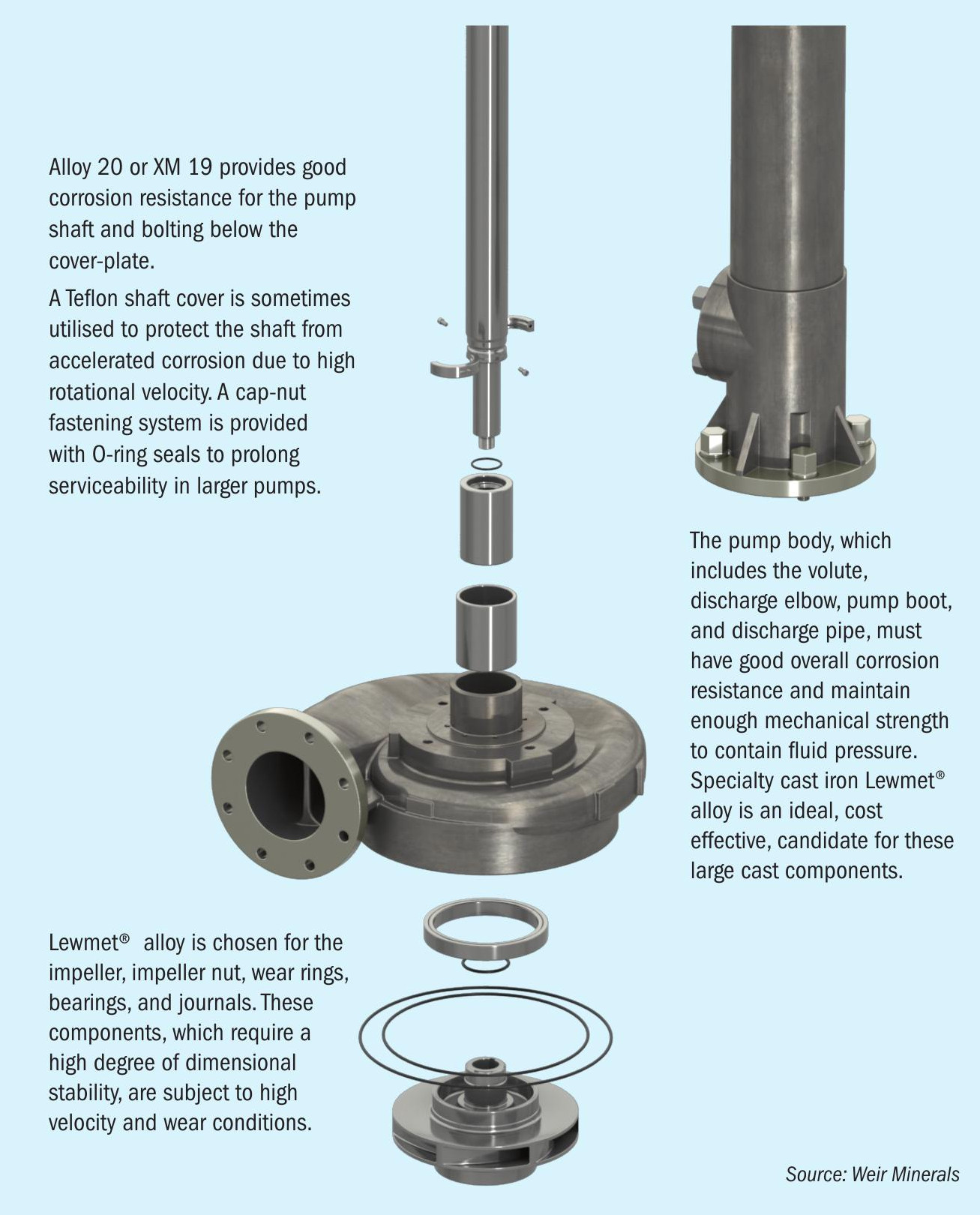
Chromium alloy metals are selected for use in high temperature heat recovery service with 99.5% sulphuric acid to 220°C. These alloys include austenitic and duplex stainless steels having high chromium content. However, a minor decrease in concentration at these high operating temperatures will cause high corrosion rates for these alloys, especially if exposed to high velocity conditions. Lewmet® 15, a proprietary high chromium duplex stainless steel of Lewis Pumps, has exhibited excellent corrosion resistance in heat recovery applications. It can be hardened to approximately 375 BHN (40Rc) and has shown superior resistance to weak acid corrosion as evidenced in process variable excursions in existing heat recovery systems. Note that high silicon stainless steels are not recommended for this service.
Selection criteria
Using a typical Lewis pump as an example (see Fig. 6), the materials of construction chosen for a given service vary according to the specific requirement of each the component parts:
Lewmet® 33/44 is chosen for the impeller, impeller nut, wear rings, bearings, and journals. These components, which require a high degree of dimensional stability, are subject to high velocity and wear conditions. Lewmet® 33/44 was specifically designed for this service.
Readily available in wrought bar form, Alloy-20 or XM-19 provides good general corrosion resistance for the pump shaft and bolting below the cover-plate. To prolong serviceability, especially in larger pumps, a Teflon shaft cover is also utilised, and a cap-nut fastening system provided with O-ring seals.
The pump body, which includes the volute, discharge elbow, pump boot, and discharge pipe, must have good overall corrosion resistance and maintain enough mechanical strength to contain fluid pressure. Specialty cast iron L-14® is an ideal, cost effective, candidate for these large cast components.
By critically assessing the most appropriate material choice for a given service condition, pumps, valves, and piping can be manufactured that not only assure adequate service life but do so in the most cost-effective manner.
References