Nitrogen+Syngas 385 Sept-Oct 2023
30 September 2023
A climate silver bullet
LOW CARBON HYDROGEN
A climate silver bullet
The CO2 emissions in a hydrocarbon fed hydrogen plant occur largely during the energy intensive syngas production step. Hydrogen production is therefore a major factor in the CO2 emission balance of an ammonia plant. BASF’s OASE® technologies for CO2 capture are capable of achieving cost-effective 99.99% carbon capture at scale. In this article Elena Petriaeva and Bernhard Geis of BASF investigate different grey and blue hydrogen production technologies.
To meet the emission reduction targets required by 2050 under the EU Green Deal, energy sources will need to shift to being almost entirely carbon-free. An intermediate milestone to support this target to reach carbon neutrality is Europe’s plan is to reduce CO2 emissions by 55% from the 1990 level by 2030. This points to a significant role for hydrogen, which can be produced via low-carbon routes from electricity or with carbon capture and storage (CCS). Moving forward, the use of pure hydrogen (H2 ) can support the energy transition while the high purity captured carbon dioxide (CO2 ) can be directly sequestered or utilised to manufacture chemical products for commercial scale.
It is widely discussed that blue, or low carbon hydrogen, and green hydrogen should be used to decarbonise the energy sector. However, a clear definition is yet to be developed. Green hydrogen has great potential, but it is not ready to cover market needs. Nearly 96% of all hydrogen is derived from fossil fuels, with natural gas being by far the most frequently used with an estimated 49%, followed by liquid hydrocarbons at 29%, 18% from coal and about 4% from electrolysis and other byproduct sources of hydrogen3 . Given that there are a lot of existing grey hydrogen plants and with the current affordable cost of fossil fuels in many parts of the world, blue hydrogen is the most economical transition technology to achieve large CO2 emission reductions.
Technologies for sustainable (blue) hydrogen production
Most of the hydrogen, ammonia, and methanol plants in operation worldwide use conventional steam methane reforming. In the steam reforming process, carbon dioxide is produced as a by-product and emitted from two different sources: as part of the flue gas (FG) and as part of the process gas (PG).
Advanced technologies for synthesis gas production such as the autothermal reactor (ATR), autothermal reactor combined with a gas heated reformer (ATR+GHR) and partial oxidation (POx) are less common and have only one source of CO2 emissions, the process gas. These technologies have already achieved large scale industrial operation and can be used for many different products including ammonia. By having only one source of CO2 emissions, both the highest carbon capture rate (CCR) and lowest opex can be achieved. It is always easier to retrofit existing grey hydrogen plants with CCS to make them blue to achieve a smaller carbon footrprint than building a grassroot green plant.
Steam methane reforming (SMR)
Conventional hydrogen production based on steam methane reformer (SMR) technology without any CO2 reduction mechanisms leads to emissions of approximately 10 kg CO2 per kg H2 depending on the feedstock and site-specific set-up. Hydrogen produced in this way is called grey hydrogen6 .
An international standard for blue hydrogen is not yet established. Decarbonising flue gas has in most cases a practical target of 90% CO2 capture. The possibility to rearrange process layouts and efficiently remove pure CO2 from synthesis gas, as well as more efficient flue gas capture units has changed the practical/feasible limit to 90-99% capture. Carbon capture is driven by the expectation of a CO2 tax on emissions and the possible income from selling the captured CO2 .
SMR without CO2 capture (grey H2 )
Producing hydrogen from hydrocarbon feed inherently results in CO2 , which must be removed as part of the process. The hydrocarbon feed is converted to a synthesis gas comprising H2 , CO, and CO2 . The CO then passes through a water gas shift reactor where it shifts with H2 O to form H2 and CO2 , resulting in a synthesis gas comprising mostly H2 and CO2 .
CO2 can be recovered from two streams in typical SMR scheme: from the high-pressure syngas stream upstream of the H2 purification step (pre-PSA syngas) and from the reformer furnace flue gas stream. Between 50 and 85% of the total CO2 emissions from a hydrogen plant are contained in the syngas stream. If more extensive CO2 recovery is desired, one can also recover it from the flue gas stream, but due to low stream pressure and low CO2 concentration, this type of recovery is more complex and more costly.
One of the more widely used CO2 removal systems is based on scrubbing with amine solvents. BASF’s OASE® white is an industry leading proven amine scrubbing technology for deep CO2 removal from syngas and offers great energy efficiency and robust operation, achieving targeted process gas CO2 capture rate of up to 99.99 mol-%.
For flue gas carbon capture, OASE® blue technology was developed specifically as an optimised post-combustion capture technology with low energy consumption, low solvent losses and an exceptionally flexible operating range. OASE® blue for flue gas CO2 emissions capture and OASE® white for process gas CO2 emissions capture are demonstrating that by combining two effective technologies the target of the lowest overall CO2 footprint is achieved.
SMR with process gas CO2 capture (blue H2 )
If the carbon dioxide in the syngas (process gas CO2 ) is captured and stored or sent for further usage roughly 60% can be captured. The consortium CertifHy has defined a threshold of CO2 intensity, below which the hydrogen is labelled “blue” or “low carbon” hydrogen: 36.4 g CO2 eq/MJ H2 (LHV). This threshold is under discussion to become more stringent1,2 .
It can be typically achieved with a natural gas (NG) based steam reformer (SMR) without major process adaptions except a CO2 capture unit installed on the syngas, from which about 50 to 85% of the total CO2 emissions can be captured. A block scheme with CO2 capture (BASF’s OASE® white) from the process gas is shown in Fig. 1.
The exact process configuration depends on the partial pressure of the acidic components in the feed gas and on the treated gas specification. The choice of process configuration depends on the design philosophy. It can be optimised based on capital expenditures (capex) or on operational expenditures (opex). The local availability and the cost of thermal energy play an important role in the choice of the process configuration.
The specific thermal energy consumption of an OASE® white unit in an SMR configuration is 1.2 GJ/t CO2 for an energy-efficient configuration (minimum opex) and 2.0 GJ/t CO2 for a minimum capex configuration at a carbon capture rate of 99.99%8 .
SMR with flue gas CO2 capture (blue H2 )
Fig. 2 is a block scheme of a typical system for CO2 removal from steam reformer furnace flue gas. The OASE® blue CO2 capture unit is based on a conventional design comprising an absorber and stripper configuration. The basic principles are the same as for the recovery of CO2 from a high-pressure syngas stream, however CO2 recovery from flue gas has several additional challenges over that of syngas, which makes the unit’s design and operation more complex and more expensive.
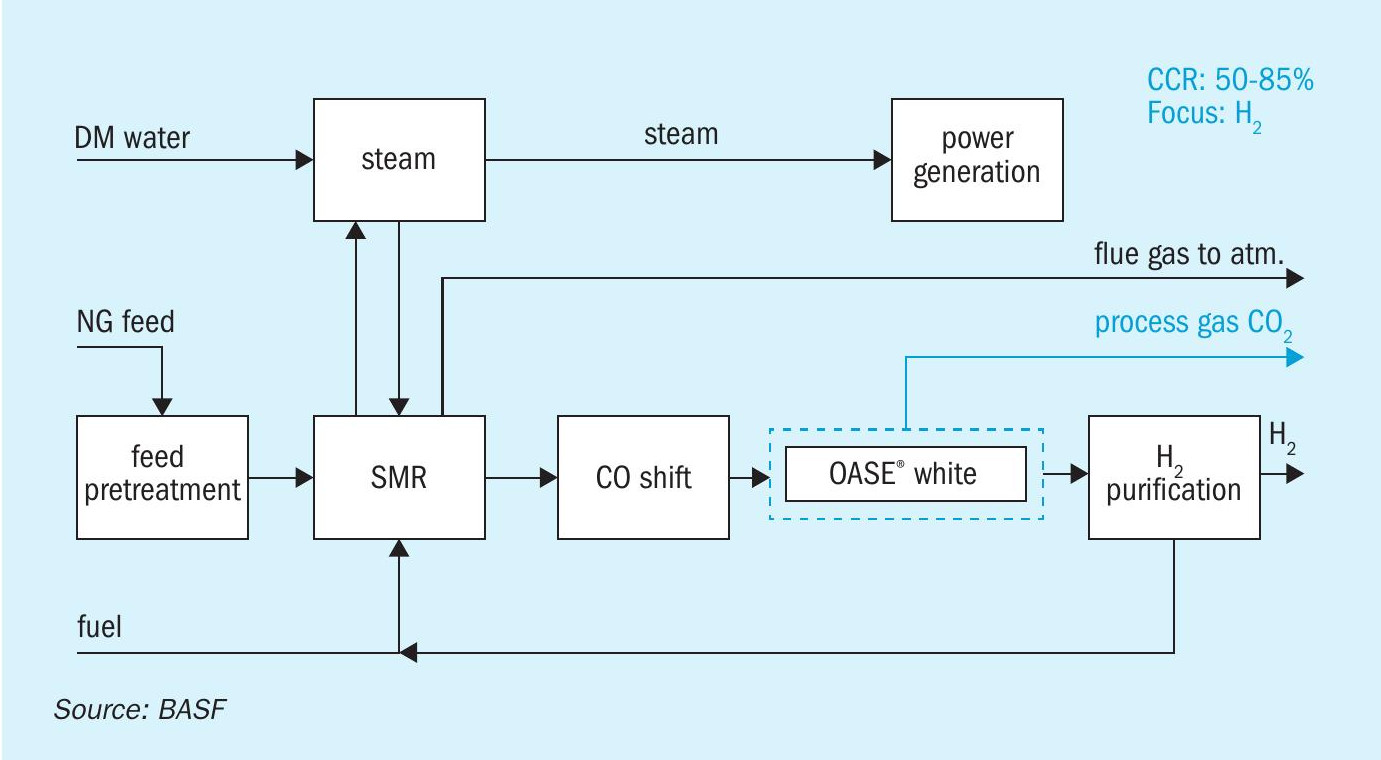
Additional equipment is required for the flue gas capture in comparison to the process gas capture. One main advantage is that the process removes CO2 from flue gas without disturbing the upstream pressure or operation of the SMR process. Before the flue gas enters the absorber, it often has to undergo various pre-treatment stages. These comprise e.g., denitrification (DeNOx), desulphurisation (SO2 /SO3 ), dust removal by a wet electrostatic precipitator or filter bag house, or a direct contact cooler (DCC). Since the gas is at atmospheric pressure, compression is required before further processing. Low pressure leads to larger equipment size. The presence of oxygen and water leads to increased rate of solvent degradation and equipment corrosion, necessitating special solvent formulations and specific metallurgy.
The specific thermal energy consumption of an OASE® blue unit in an SMR configuration is 2.56 GJ/t CO2 at a carbon capture rate of 95%8 .
The process configuration mainly depends on the CO2 concentration in flue gas, the CO2 production rate and economics and customer preferences. The decision on the process configuration selection is a trade-off between capex and opex. BASF offers various advanced integrated schemes which lead to further reduction of specific thermal energy requirements. The availability and cost of thermal energy may play an important role especially in larger plants, while in smaller units sometimes a bare bones design philosophy is optimal.
All these factors make low-pressure flue gas CO2 recovery more expensive compared to the high-pressure syngas option. At times, for cost savings or to limit the amount of CO2 recovery (due to market, storage limitation, or other conditions), the amine unit can be installed only on part of the total stream, with the rest of the stream by-passing the CO2 recovery unit4 .
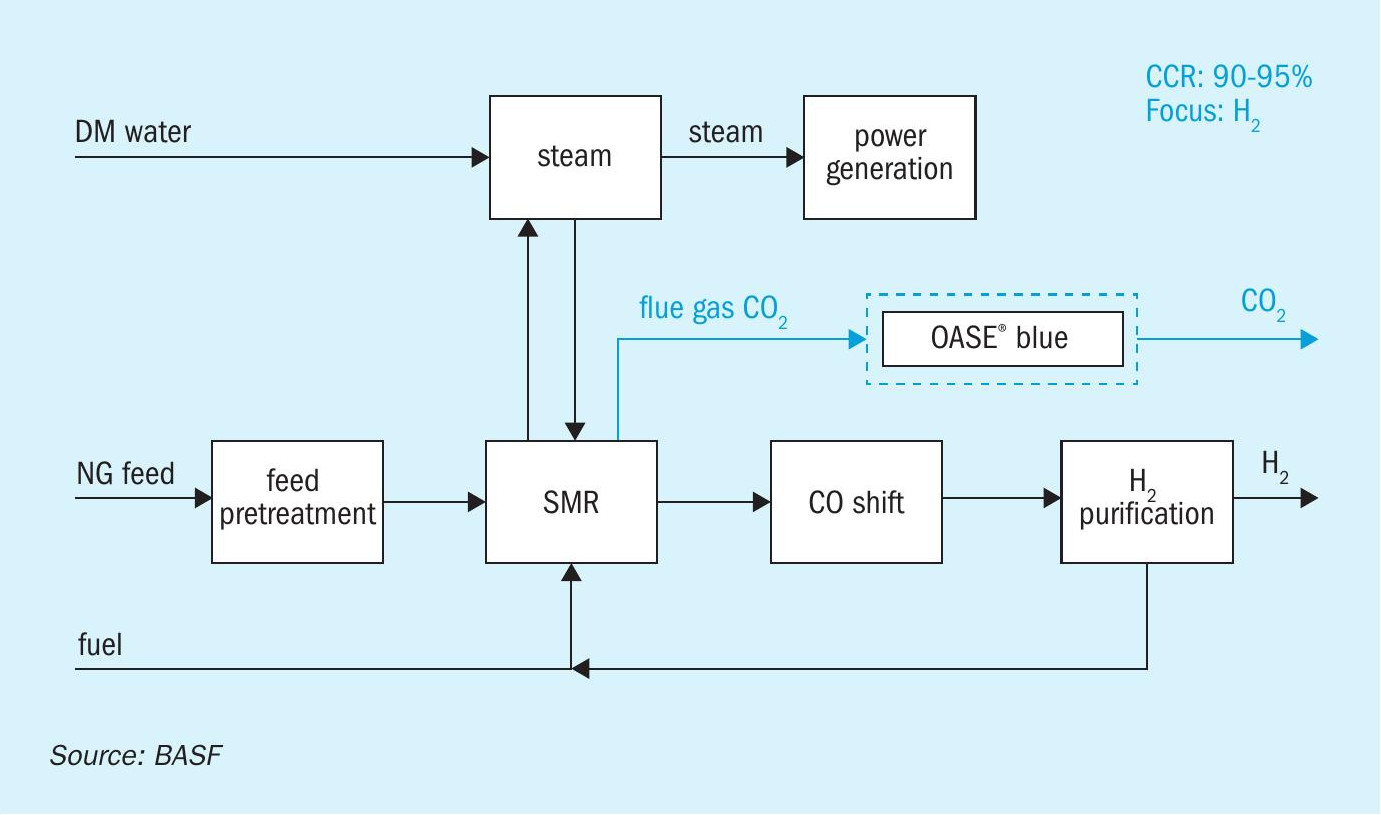
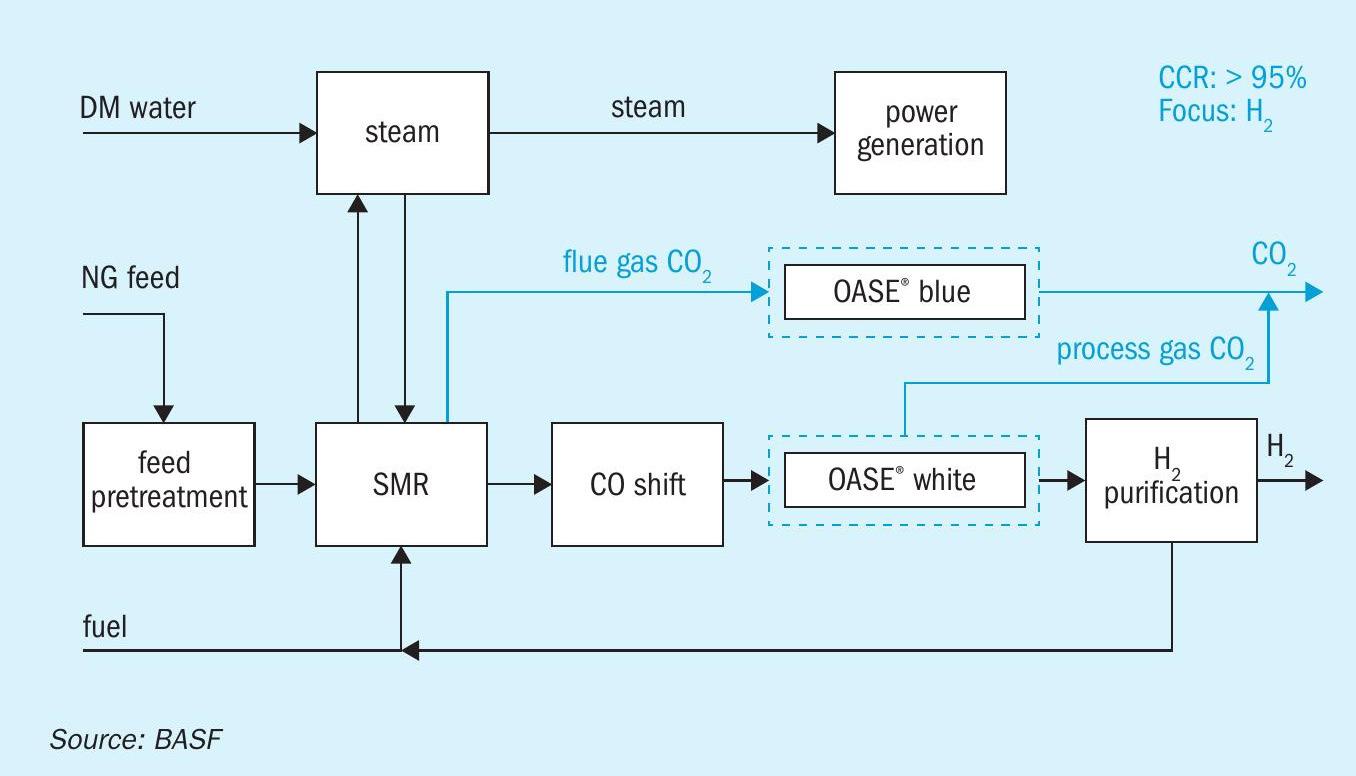
SMR with process gas CO2 capture (e-furnace) (blue H2 )
One of the most recently developed improvements in steam reforming technology is an electrically heated steam furnace using electricity from renewable sources. This fundamentally new approach has the potential to reduce CO2 emissions by 98%. The demonstration plant construction has been started and the plant is to be fully integrated into one of the existing steam crackers at BASF’s Verbund site in Ludwigshafen, Germany.
The specific thermal energy consumption of an OASE® white unit is the same as previously mentioned for the SMR configuration.
SMR with process/flue CO2 capture (blue H2 )
By capturing CO2 from both sources in a typical SMR-based hydrogen production technology, from syngas preparation and from the flue gas emitted from steam reformers and fired heaters, it is possible to achieve a CCR of more than 95% (see Fig. 3).
The specific thermal energy consumption for OASE® white and OASE® blue units along with achieved CCR is the same as previously mentioned.
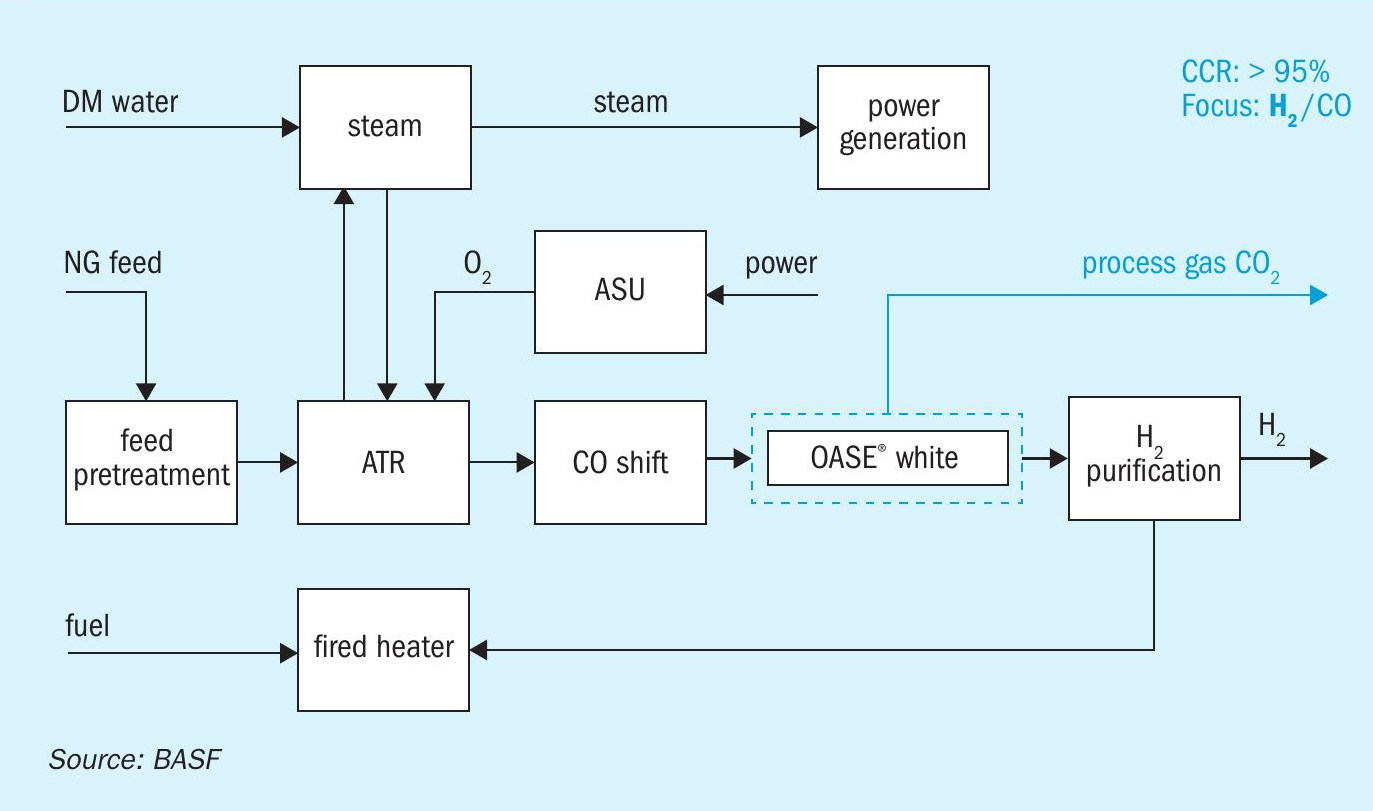
Although the majority of hydrogen is currently produced by SMR, being the most proven and reliable technology for hydrogen production, the described schematics might fit better for SMR retrofitting and converting grey into blue or low carbon hydrogen/ammonia. For grassroot units, advanced technologies might be the best choice in terms of economics by providing the lowest capex designs at high production capacities, however, this requires installation of energy-intensive air separation units (ASUs) and onsite handling of oxygen which has several safety risks that need to be mitigated. The decision of which hydrogen production technology to select depends on customer preference, experience, and availability of energy resources at site.
Advanced technologies for hydrogen production
Within the hydrogen industry new plant production capacities are constantly increasing. This exceeds the economic limits of steam reformer technology and therefore advanced hydrogen production technologies have generated interest. Additionally, large-scale single trains bring economy of scale. The synthesis gas generation technologies using auto-thermal reforming and partial oxidation are operating with a reduced steam-tocarbon ratio below 1.0. This reduces the amount of heat input for the reforming section drastically, and thereby the fuel requirement directly leading to lower CO2 emissions.
Therefore, when decarbonising, the use of any of the advanced technologies for hydrogen production is beneficial since they either require a smaller or no flue gas capture unit or less hydrogen for fuel, which is a major advantage when compared to steam reformer-based solutions6 .
Technology transition from conventional SMR-based technology to advanced technologies, such as auto-thermal reforming (ATR), partial oxidation (POx) and an autothermal reformer + gas heated reformer (ATR+GHR) are described below. Selection of technology is defined by the required product: hydrogen, hydrogen with carbon monoxide or carbon monoxide with hydrogen.
ATR with CO2 capture (blue H2 )
Autothermal reforming (ATR) is an advanced technology for hydrogen production where oxygen, carbon dioxide and steam react with methane, producing syngas. ATR combines steam reforming and POx resulting in an overall reaction enthalpy close to zero. The process consists of a POx zone, which supplies the process heat for the subsequent endothermic steam reforming step using catalysts. The total heat balance and the H2 /CO ratio in the product gas are adjusted by varying values of S/C and O/C5 .
ATR has a higher hydrogen yield than POx and provides more flexibility in terms of process conditions, start-up time, and complex feedstock utilisation than the SMR. ATR in principle is used as a secondary reformer in all ammonia production units where high H2 /CO ratios are needed. In the case of ammonia production, compressed air is used instead of oxygen. Autothermal reforming is installed in ~5% of all hydrogen and methanol production units by using oxygen from an air separation unit.
Fig. 4 shows a typical ATR scheme with the option of process gas CO2 capture.
The specific thermal energy consumption of an OASE® white unit in an ATR configuration is 0.8 GJ/t CO2 for a minimum opex configuration with a carbon capture rate of 97%.
ATR+GHR with CO2 capture (blue H2 )
The autothermal reformer + gas heated reformer (ATR+GHR) configuration has been developed to maximise energy recovery. The main difference between ATR+GHR and SMR is that the energy used to drive the steam reforming reaction is provided by introducing oxygen to the ATR rather than burning natural gas in SMR. In this case oxygen is obtained from an air separation unit (ASU) and by-product nitrogen could be utilised at site7 .
ATRs are already used in the production of syngas and are an integral part of most modern schemes for production of methanol and for synthetic liquid fuels from Fischer-Tropsch processes. These plants are very large and demonstrate that the technology can produce hydrogen at large scale, thereby eliminating scale-up risk. GHRs have operated on a commercial basis for over 100 cumulative plant years and have demonstrated reliability7 .
The specific thermal energy consumption of an OASE® white unit in an ATR+HGR configuration varies between 0.69 and 1.1 GJ/t CO2 , depending on configuration along with an achieved carbon capture rate of 99.99%.
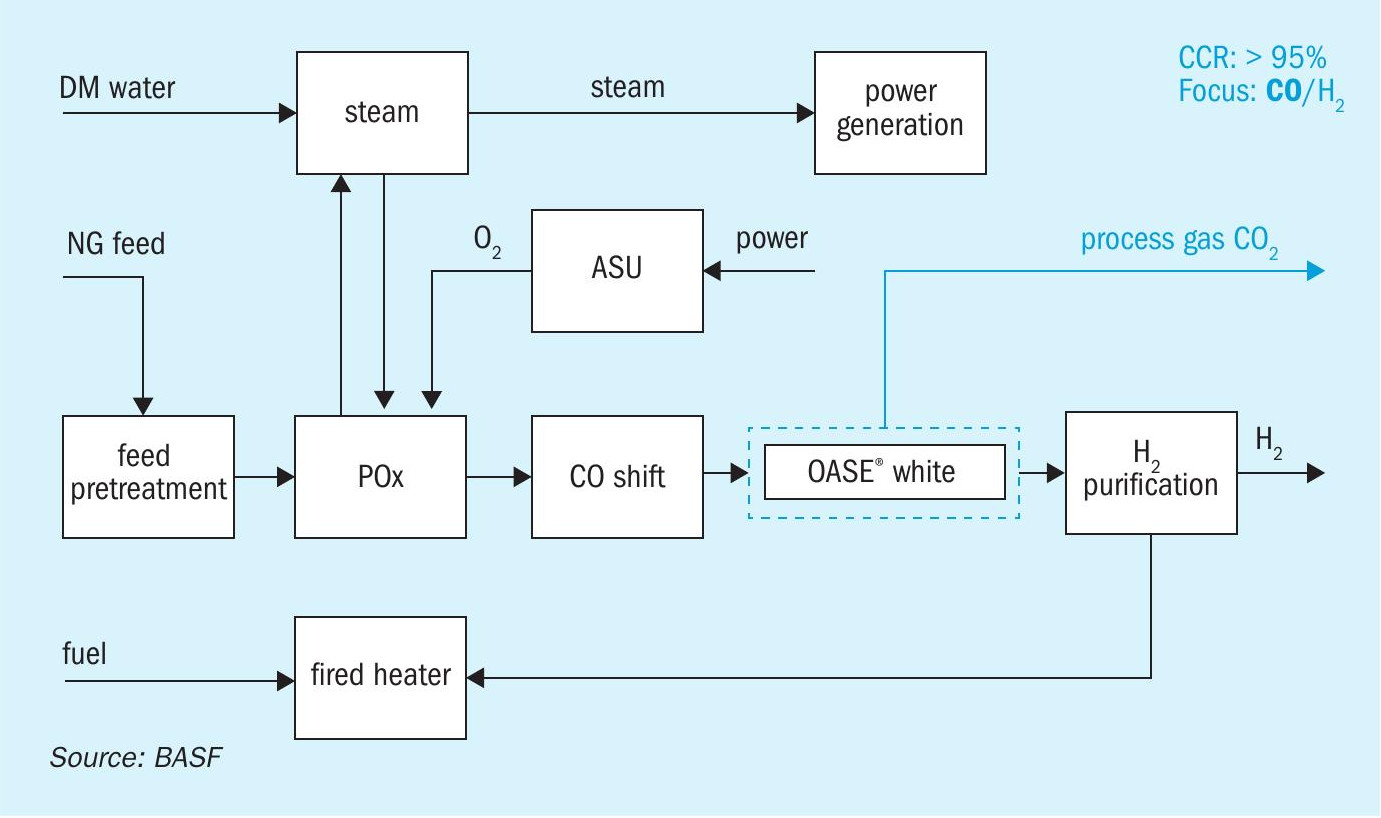
POx with CO2 capture (blue H2 )
Partial oxidation (POx) is an oxygen-based, non-catalytic technology which is a mature and cost-efficient process used by ~10% existing hydrogen plants. Being non-catalytic, unlike SMR, ATR and ATR+GHR, POx doesn’t require the same extent of gas pretreatment as catalytical processes and can still provide significant feed flexibility. The POx design (Fig 5) has a lower hydrogen yield than ATR.
Fig. 5 shows a typical POx scheme with the option of process gas CO2 capture.
The specific thermal energy consumption of an OASE® white unit in POx configuration is 0.6 GJ/t CO2 along with a carbon capture rate of 99.99%.
By having the highest CO2 partial pressure in the feed gas, this configuration provides the lowest specific energy consumption in comparison to other options.
Conclusion
The demand for hydrogen is accelerating, driven by stronger national-level government commitments to decarbonise the energy sector and by businesses with net-zero objectives and ambitious sustainability targets. Meeting this demand will require a transformation in how hydrogen is being produced. Just as there is no magic bullet for providing the world’s green energy needs, there is no one-size-fits-all approach to clean hydrogen production. Current economics of green hydrogen are challenging when compared to blue hydrogen. Blue hydrogen has a vital role to play in energy transition and is needed in the short and medium term.
BASF’s OASE® technologies for CO2 capture have been independently assessed to be the most cost-efficient and are capable of achieving up to 99.99% carbon capture at scale in various hydrogen production flow schemes. BASF being not just a technology provider and licensor, but also a facility owner and operator of its own units with a commitment to reducing its impact on the climate, understands the challenges and concerns of operating companies around the globe. BASF regards blue hydrogen as a timely climate silver bullet, providing a more cost-effective alternative to green and carbon-intensive grey hydrogen.
References