Nitrogen+Syngas 386 Nov-Dec 2023
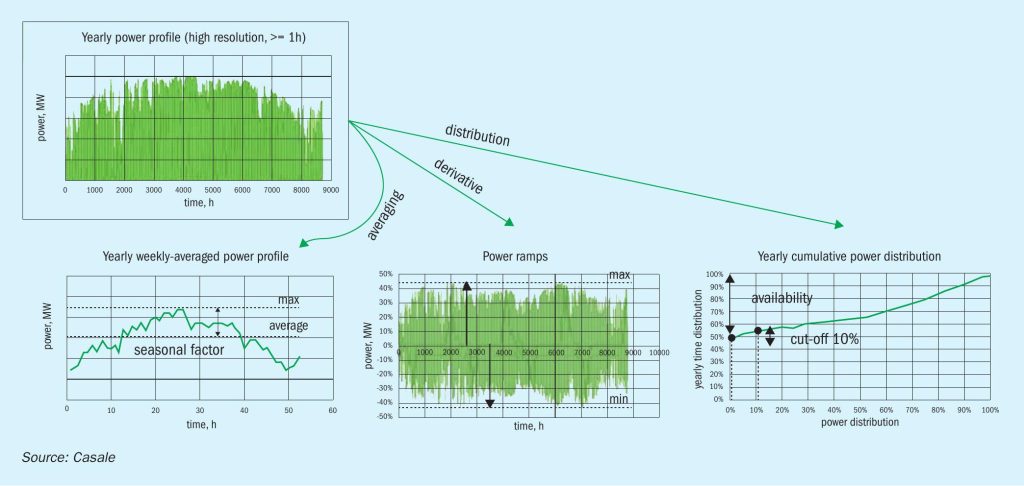
30 November 2023
Ammonia plant hybridisation
The ammonia industry is expected to change drastically in the coming years to meet sustainability goals and to face the problem of climate change. New low carbon ammonia plants as well as fully green facilities are expected to be commissioned to meet the target of climate neutral production. The integration of an existing ammonia facility with green hydrogen to supplement or replace the grey ammonia production with green ammonia represents a low-risk solution to meet the requirement for running clean ammonia plants and offers the most competitive green ammonia production cost in the short term. In this article Sergio Panza and Marco M. Carlucci of Casale paper present different scenarios based on energy availability at battery limits.
The energy transition is pushing industries toward a more sustainable model where old plants are upgraded, or converted with new technologies and processes, to reduce their environmental impact.
Increasingly stringent emissions regulations and ambitious zero-carbon energy goals are also moving existing players of the fertilizer industry as well as new investors of the ammonia industry to design new plants to meet the goals set by the energy transition model.
New plants alone cannot meet the targeted climate neutral production of goods in the timescale required and so a strategy must be developed for the approximately 600 fossil fuel based existing ammonia plants which need to be upgraded in order to satisfy new climate requirements.
Carbon border tax mechanisms are also going to play a crucial role for export-oriented producers in order to provide a more competitive product on the market. The demand for low carbon ammonia will be facilitated by end users driven by a carbon reduction strategy to demonstrate their compliance with a societal demand toward a zero-carbon goal.
Plant revamping to reduce the specific energy consumption and the CO2 emission in general is one possible route towards this goal. Another option is plant conversion to a blue configuration with carbon capture and utilisation (CCU) or carbon capture and sequestration (CCS).
Another interesting route to update existing plants to a configuration with a lower environmental impact is the so-called hybridisation route, where green hydrogen produced by electrolysers is integrated in the existing facility with the target to decrease the CO2 footprint and potentially to increase the plant ammonia production. Renewable energy can be utilised to promote such green hydrogen production allowing the partial (or complete) production of a premium product (green ammonia).
Planning for the continued hybridisation of existing plants should be done and will require investments enabling the transition from grey to green ammonia production.
The European renewable energy directives are pushing producers to the hybrid revamp of their existing ammonia plants, in order to reduce the carbon taxation and possibly fetch a premium price for the low ammonia carbon production.
In hybrid plants the green ammonia production cost will be quite competitive, as the capex is greatly reduced by the utilisation of many of the existing sections such as the ammonia synthesis capacity, and existing utilities.
Depending on the type of energy supply to the electrolysers (steady or erratic mode), and depending on the extent of plant hybridisation (percent of green ammonia production compared to the overall one), different outcomes are expected for the existing ammonia plant.
Casale solutions are tailored to client requests and consider such revamping boundary conditions.
Degree of hybridisation
Depending on the extent of green hydrogen integration in the existing plant and the type of green hydrogen supply (steady or erratic), different outcomes and modifications could be required to implement the new hybrid arrangement.
In general, green hydrogen is injected at the suction of the syngas compressor machine even if other locations are also possible (e.g., upstream of the methanator or directly inside the synthesis loop).
In case green hydrogen supply contains potential poisons for the ammonia converter catalyst (e.g., oxygenated compounds, sulphur compounds, halogens, olefins), adequate pre-treatment must be foreseen before integrating it into the existing plants.
For a low degree of hybridisation (say green hydrogen represents less than 5-10% of the total hydrogen fed to the ammonia synthesis), it is reasonably expected that no (or minor) modifications are necessary to the existing ammonia plant, regardless of the type of green hydrogen supply (steady or erratic).
Steady power supply
If the renewable source is always available at constant value (e.g., from hydroelectric or geothermal power), a high degree of hybridisation of the existing plant can be reached with few modifications.
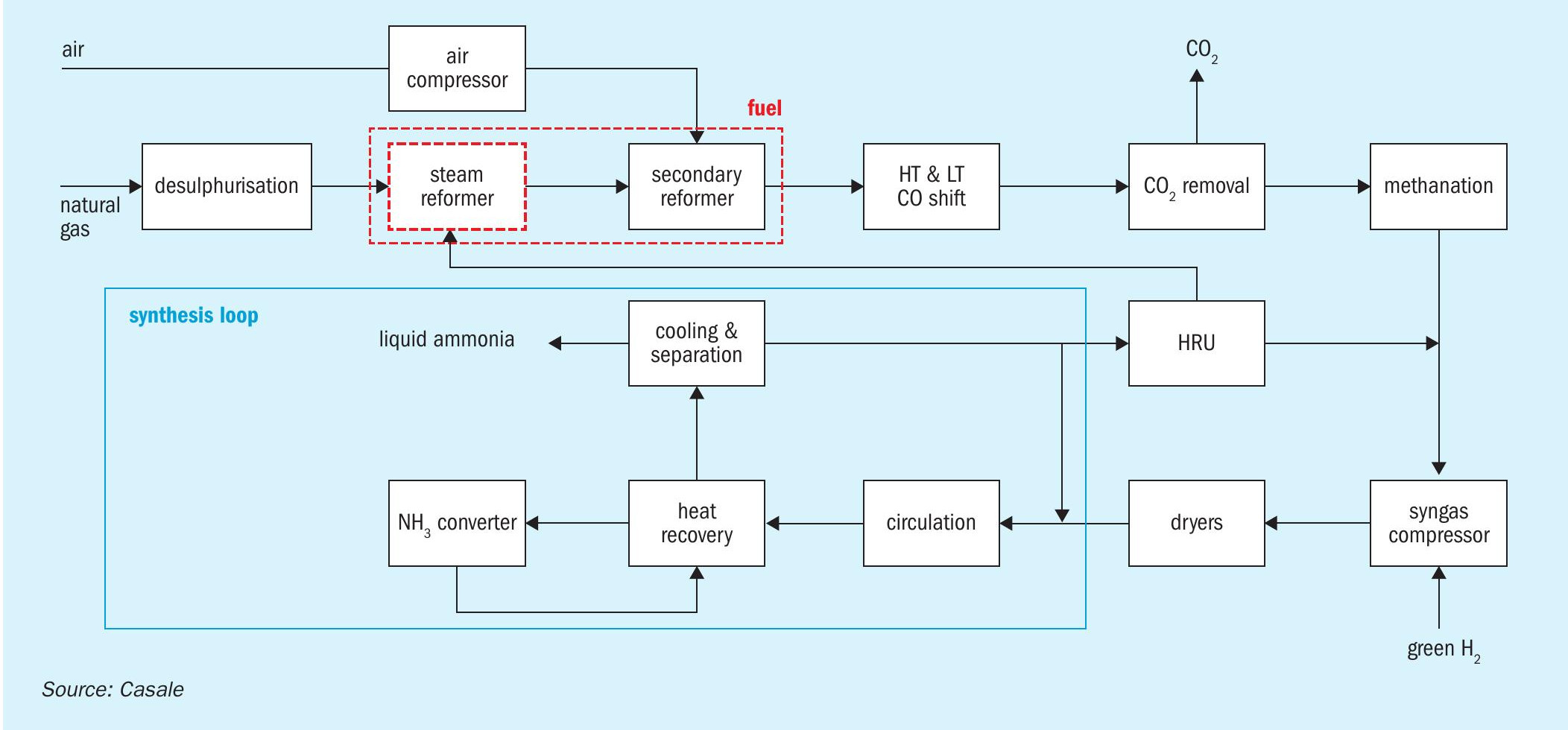
Low plant hybridisation (typically up to 10%) with steady green hydrogen supply can be accommodated without any major modification.
Total hydrogen supply at the syngas compressor suction must deal with existing machine limitations, so it is necessary to reduce the front-end load to accommodate the extra gH2 .
Since part of the hydrogen is supplied at the syngas compressor suction, bypassing the plant front-end, a change in the operating conditions of the reforming section is expected. In particular, the air to feed gas ratio in the secondary reforming section needs to be increased to compensate for the extra hydrogen and to supply the required nitrogen.
To control the secondary reformer outlet temperature and avoid jeopardising the downstream waste heat boiler, the primary reformer outlet temperature is decreased. The methane slip of the reforming section is controlled as duty is shifted from primary to secondary reforming.
The impacts on the primary reformer convection coils should be evaluated, but typically at 10% hybridisation they are minor and no modification to the convective section is required.
Changes in the steam balance are also expected due to the different boiler production at the new operating conditions.
The synthesis loop and in particular the ammonia converter operating conditions could benefit from green hydrogen integration thanks to the lower inert content of the new makeup gas: gH2 stream from electrolysers is inert-free, allowing for more room in the plant back end.
The lower inert content requires a careful check of the outlet temperature from the ammonia converter, to avoid any operation of the converter and the downstream equipment beyond their temperature limits.
Plant hybridisation will potentially reduce the CO2 production in the process line, however in case of a CO2 requirement for downstream production (e.g., for a urea plant), the process gas flow through the reforming section can be kept unchanged, recycling part of the synthesis gas downstream of the CO2 removal section to the fuel gas system.
In this way a pre-combustion strategy for carbon capture can be integrated with green hydrogen injection into the plant.
Another revamping option, in case of the availability of an oxygen stream from electrolysers and steady state integration, is the injection of the oxygen stream into the process air going to the secondary reformer to enhance the methane conversion in the secondary reformer.
Since the load of the primary reformer radiant box is further reduced, the amount of flue gas going to the convection section is also decreased, allowing for additional plant CO2 emissions reduction.
The primary reformer convective section should be verified since the operation of the convection coils and steam superheating in particular will also be affected.
As previously indicated, a low degree of green hydrogen integration is not going to require any change to the plant configuration; conversely, a larger integration would involve a deeper check of the existing plant and likely involve some modifications. However, such plant upgrading can be treated as a “standard” revamping project, due to the stability of green hydrogen feed.
If a mild or high degree of hydrogen integration is required (up to 30-40% and above) an external nitrogen source is necessary. The primary reformer operating temperature would be decreased too much to accommodate the required air to gas ratio, leading to fuel maldistribution in the radiant box.
Extra nitrogen feed, if not already available at plant battery limits, can be provided by a new PSA or ASU.
With enough extra-nitrogen and steady gH2 supply, a complete plant conversion to green ammonia production is achievable (i.e. 100% hybridisation). In such a case the plant front-end should be idled and ammonia production will rely entirely on gH2 and gN2 .
Full hybridisation of a plant can be reached with a step-by-step approach, taking advantage of the increasing level of CO2 taxation to improve project economics.
In case of fluctuating power supply, and therefore erratic green hydrogen feed flow to the synthesis loop, a different approach is required to avoid cyclic plant operation that would lead to fatigue issues, which could severely impact the mechanical integrity of equipment and therefore require costly upgrading interventions.
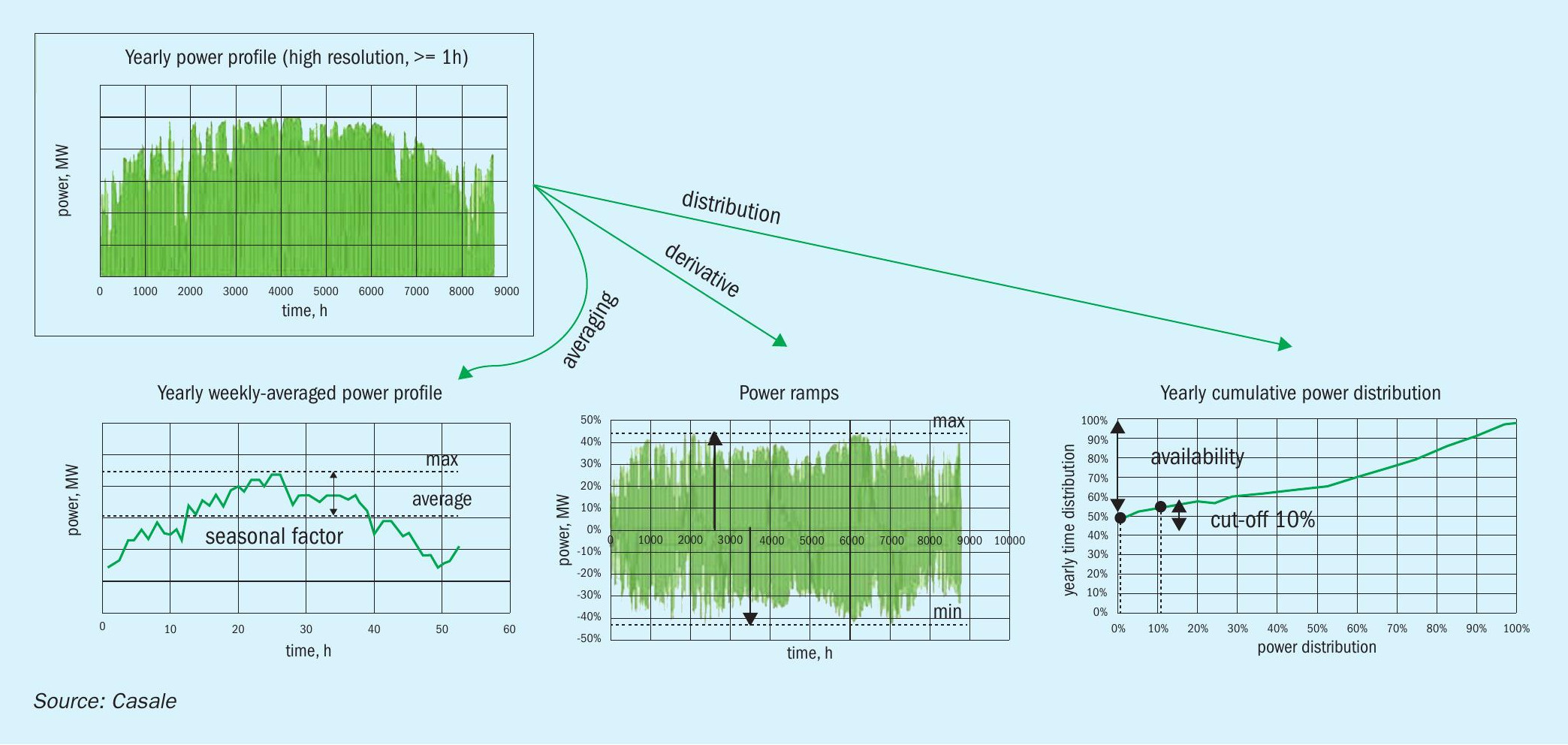
Erratic power supply
Per definition, renewable energy is often tied up with erratic power generation. Green hydrogen and green ammonia production need to be able to cope with such feed flows.
The intermittency of renewable power requires a higher flexibility of the ammonia process and presents challenges due to the intrinsic operational discontinuities.
Efficient utilisation of the available renewable power affects the project capital investment cost. The licensor goal is to minimise any impact on the capex, maximise the renewable power utilisation and ensure safe plant operation avoiding any damage due to fatigue issues.
Power profile analysis
The power profile from renewables (used to feed the electrolysers) features specific parameters that affect the design of the green ammonia plant. These parameters are:
- Capacity factor, defined as the ratio between the average production and installed capacity, which gives a benchmark for the generation over the year.
- Seasonal factor, defined as the ratio between the average of the daily average power and the maximum daily average peak. This parameter provides a better understanding of how big the fluctuations are between different seasons.
- Ramp up/down, defined as the load variation in one hour with respect to the average capacity, which gives an indication of the stability of the renewable sources.
- Availability, defined as how long a certain percentage of the installed capacity is available.
- Cut-off, defined as the renewable power that cannot be used or stored. This parameter must be minimised in order to reduce inefficiencies.
The power profile variability and fluctuation can give a preliminary idea of the economic feasibility for the project, since high variation and low availability lead to increased hydrogen storage requirements (when its use is foreseen) with detrimental effects on the capex.
As previously mentioned, a low degree of plant hybridisation (typically up to 5-10%) is not going to require major plant modifications even if the gH2 supply is erratic.
However, mild plant hybridisation (up to 25-30% gH2 ) can be carried out if an additional nitrogen source is available. The extra nitrogen can be used to compensate for fluctuations in the hydrogen feed, avoiding modification of the air to gas ratio in the front-end.
Extra nitrogen feed, if not already available at plant battery limits, can be provided by a new PSA or ASU.
In case of no extra nitrogen availability, it is still possible to target a low degree of hybridisation, but a careful check on front-end equipment must be carried out to ensure that no fatigue issues are encountered, or alternatively the plant should be adequately upgraded to cope with such operating conditions.
For a high degree of plant hybridisation (say above 30%), the use of hydrogen storage to optimise the feed profile is typically necessary to avoid costly power cut-offs and ensure the economic profitability of the project.
Hybridisation degree achievable with erratic power supply, as well as project economics, are strongly dependent on the power supply profile: in general, high capacity factor profiles will allow a higher hybridisation degree with a lower capital investment cost.
In case of erratic power supply, oxygen from the electrolysers cannot be used in the secondary reformer to promote NG reforming as it would entail a significant change to the front-end operating conditions, creating the conditions for cyclic operation of the plant, thus fatigue issues which are costly to deal with.
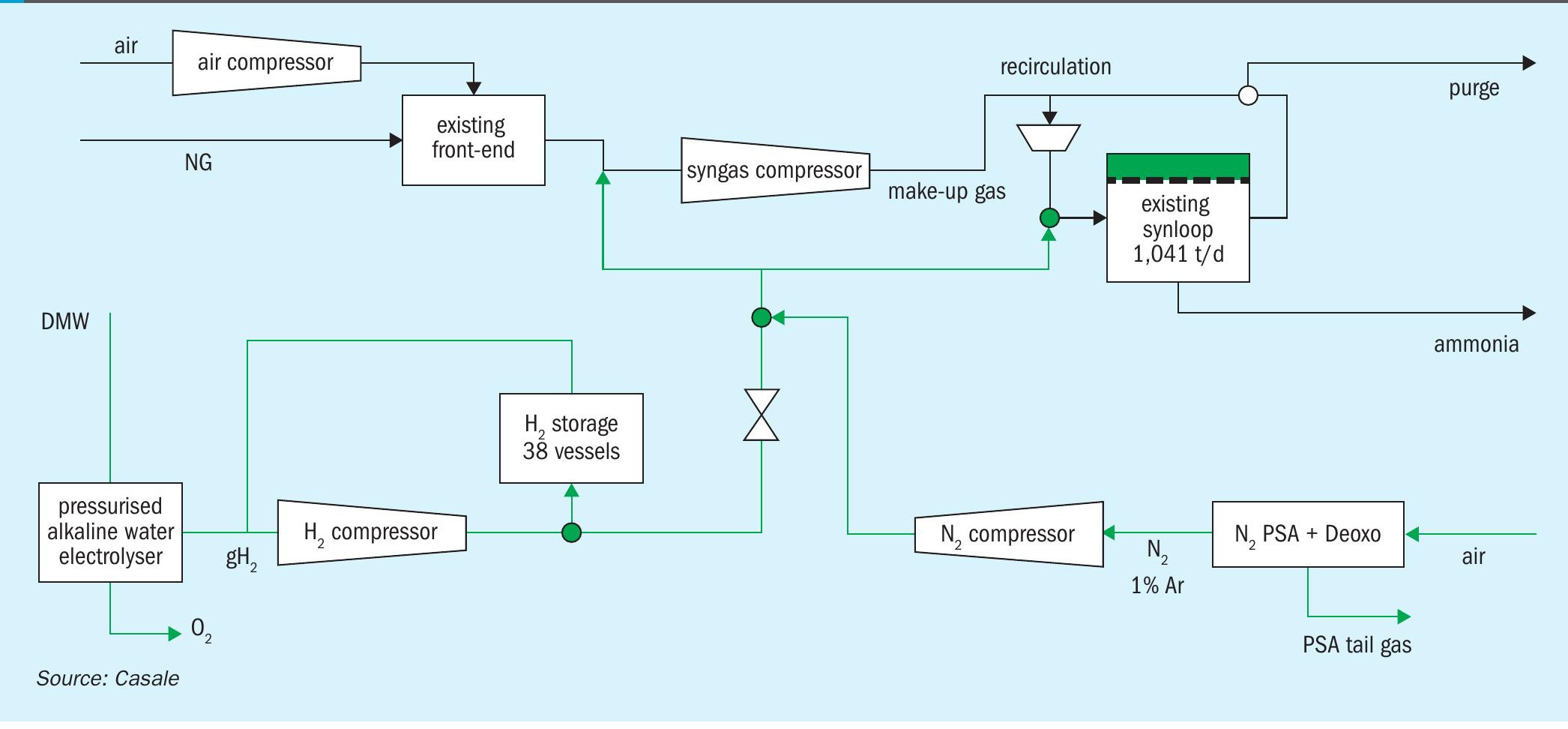
Hydrogen storage
As the electrolyser dynamic is much faster than the ammonia plant dynamic, when the amount of erratic green hydrogen supply becomes comparable to the grey hydrogen produced by plant the front-end (hybridisation degree 30% and higher), it is convenient to foresee a hydrogen buffer.
The hydrogen storage system should be designed to cope with the unpredictability of some power profiles and to increase the daily trend gH2 supply (e.g. wind and solar systems).
Operation based on so-called “hot stand-by” mode is also possible but is considered less profitable for the plant economics and is not discussed further here.
The installation of a hydrogen storage system is meant to stabilise the green hydrogen supply to the ammonia synthesis loop, maximising the gH2 intake and minimising green power cut-off.
Casale solution for hybrid plant fed by erratic power supply
Casale hybridisation of existing ammonia plants follows the below principles:
- minimise changes to the existing plant, minimise/avoid H2 storage installation;
- minimise operator engagement for daily control of plant based on input of renewables;
- minimise upset to the existing plant front-end due to the fluctuating hydrogen supply.
In case of erratic power supply: ammonia plants can typically accept 5-10% hybridisation without any major intervention. A higher degree of hybridisation (higher than 10%), as a general rule, requires some independent extra nitrogen to balance the green hydrogen.
The typical arrangement for a high degree of hybridisation (higher than 30%), erratic green energy supply and no extra N2 availability at plant battery limits is reported here, for reference.
As shown in Fig. 3 the gH2 injection can be conveniently located at the syngas compressor suction or directly inside the synthesis loop; the presence of a small hydrogen storage supply would guarantee continuous green ammonia production even when green power is not available at battery limits. The hydrogen storage would also minimise fluctuation in the hourly ammonia production.
If desired, the plant can be configured without hydrogen storage, but a small hydrogen buffer is in any case suggested to smooth green hydrogen upsets.
To minimise green power cut-off, the idea developed by Casale is to produce more grey ammonia when minimum green hydrogen is available in winter and more green ammonia in summer where maximum hydrogen production is expected.
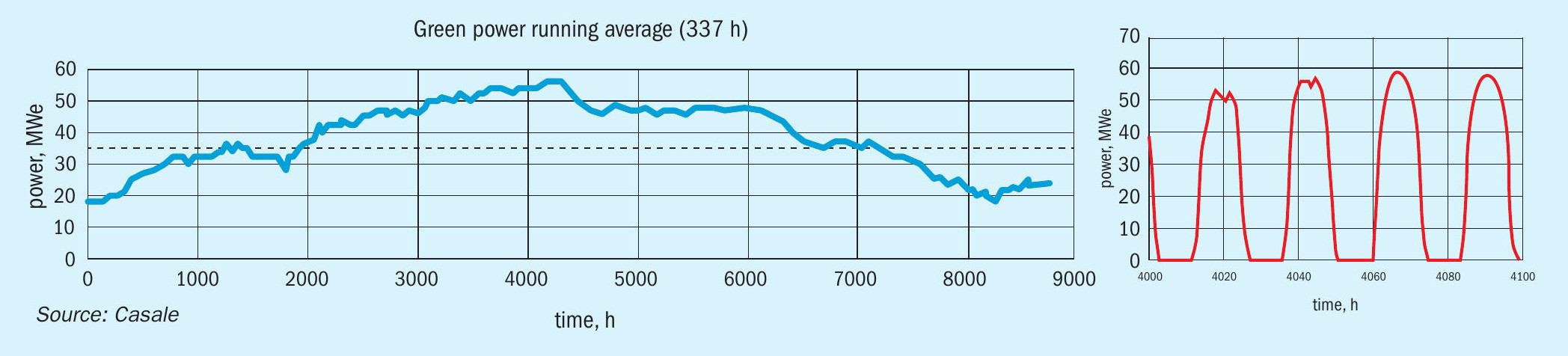
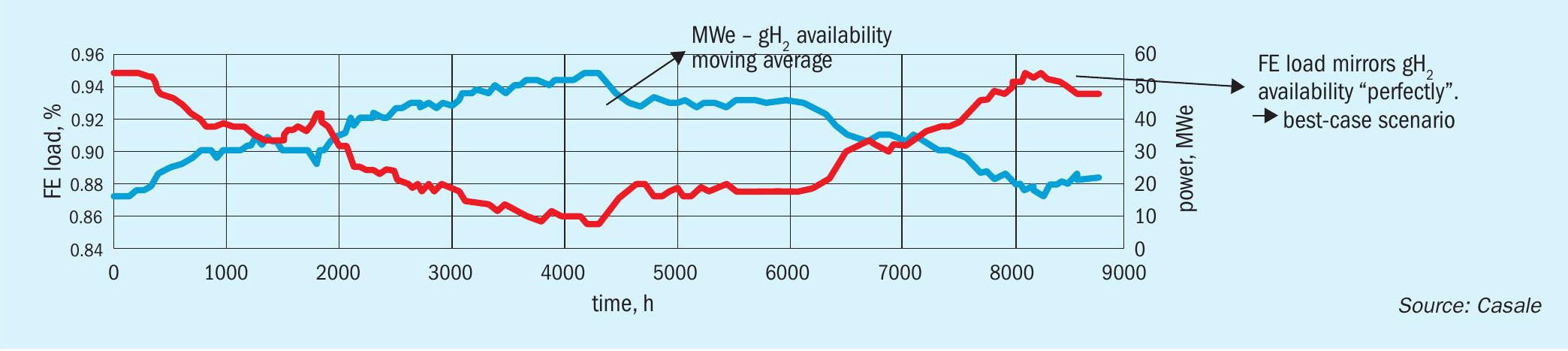
Since total hydrogen supply at the syngas compressor suction must deal with existing machine limitations, to introduce the extra gH2 it is necessary to reduce the front-end load. The final target is to achieve ammonia plant decarbonisation and potentially also a capacity increase, made possible thanks to the reduction of synloop inerts or by exploiting the room available in the existing machinery and equipment.
Considering a reference power profile such as the one in Fig. 4, the above statement can be translated in an existing frontend (FE) operation that is mirroring the green power (green hydrogen) supply (see Fig. 5).
A continuous change of the FE load following the average renewable power supply should be avoided, since this would require costly equipment upgrades to ensure their mechanical integrity due to cyclic operation of the system triggering fatigue issues.
To solve this issue, the front-end load would be modified according to a “stepped” strategy: namely the front-end load is chosen, for instance, on a weekly basis or every two weeks to avoid continuous adjustment of the operating parameters.
The back-end of the plant would be vice versa operated according to continuous and flexible adjustment of the operating parameters, according to the same philosophy applied for the Casale flexible green ammonia plant solution.
The ammonia converter would be operated keeping the synloop operating pressure and temperatures almost constant. This is achieved thanks to a properly designed control philosophy that would allow the synthesis loop to be operated over a wide range of operating load.
In Casale new green ammonia plants, for instance, such a control philosophy allows the plant to operate with a capacity range of 10-110% and according to quick ramp-up and ramp-down parameters (3%/min).
In case of power supply with a low capacity factor (i.e. low average output with respect to installed capacity) or when a high degree of hybridisation is required, the installation of a hydrogen storage system together with the aforementioned FE stepped solution becomes interesting, since it helps to maximise the green energy intake and minimise green energy cut-off.
In case no hydrogen storage is foreseen, higher back-end load variation is expected following the erratic power supply trend, in addition to higher green energy cut-off or (alternatively) lower average ammonia production.
The solution developed by Casale is based on a historical power trend, however weather forecasts can be integrated to adjust the trend to the current plant environmental conditions.
The proposed FE stepped solution offers the following benefits:
- the overall decarbonisation is almost proportional to the gH2 integration;
- FE cyclic operation is avoided;
- LCOA with this solution is lower compared to a parallel green Haber Bosch loop as well as with other solutions expecting either the use of green hydrogen as fuel or the installation of a big hydrogen storage system;
- proposed solution minimises H2 storage;
- solutions are under patenting phase (pending);
- synloop operation under erratic power supply is managed as per usual Casale green ammonia solutions (load can ramp-up and ramp-down according to Casale technology).
Hybridisation case study
To better understand the concept behind the Casale solution an example for a 1,000 t/d existing ammonia plant is presented. The selected operating case is expecting an averaged yearly green ammonia production of 10% through a plant hybrid revamp; this example can be extended to a higher degree of hybridisation providing similar outcomes.
The power generation unit is based on a full PV system as per Fig. 4, while FE load is chosen on a weekly basis as per Fig. 6.
The following example has been developed assuming to limit the plant hourly production variation within 5% between the maximum and the minimum achievable by the hybrid plant and according to the available power profile; such boundary conditions have been kept throughout the year of operation and for this reason a small hydrogen storage unit has been required.
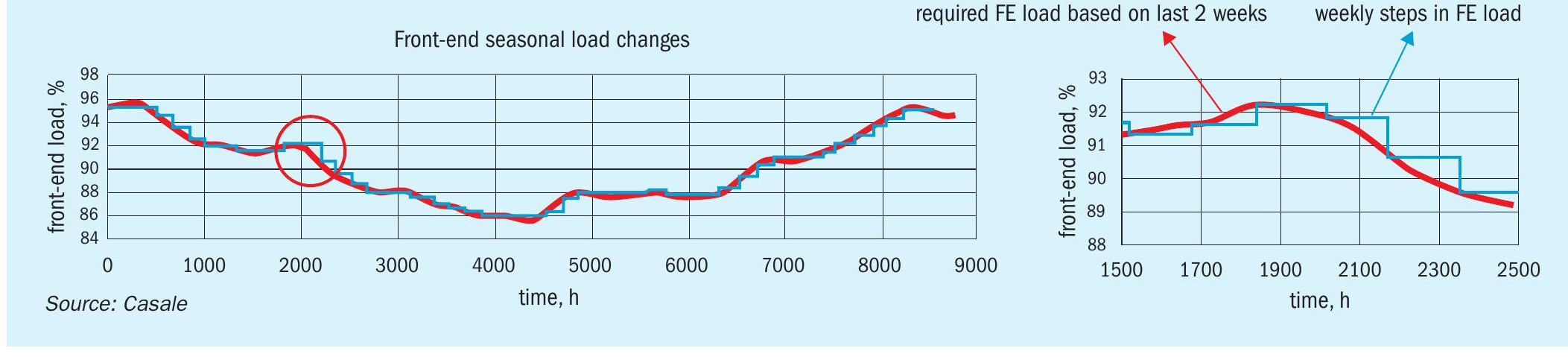
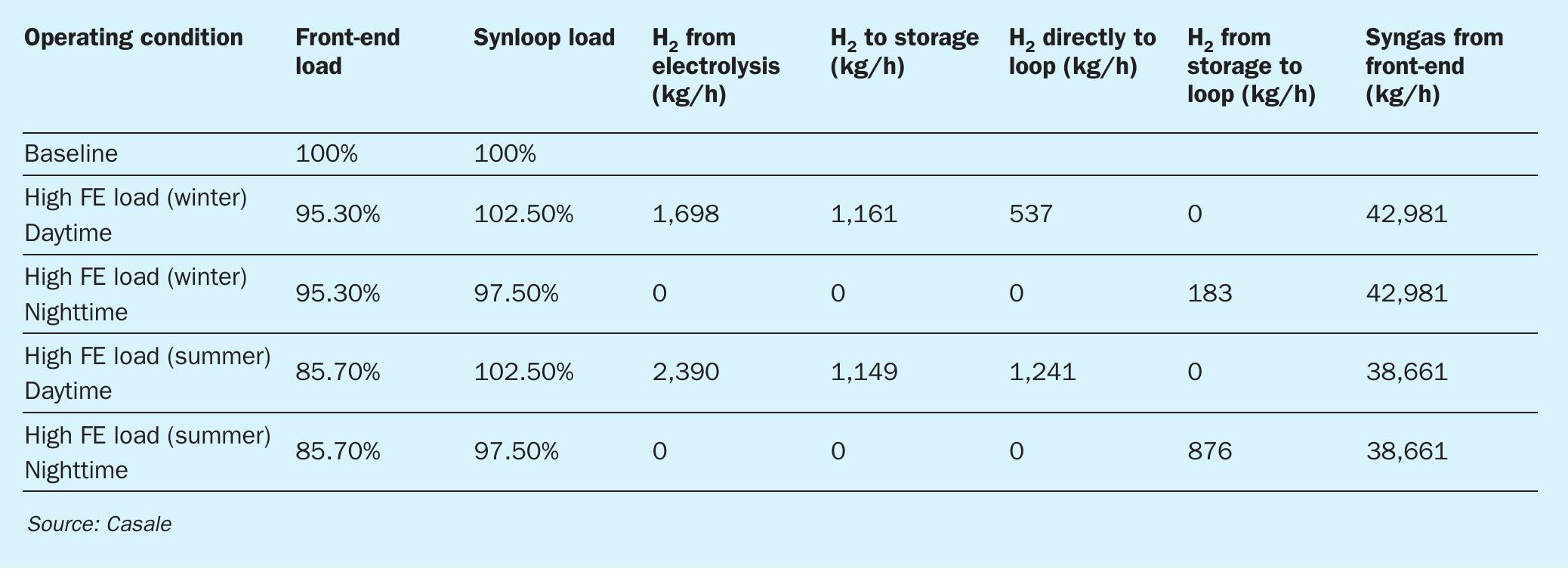
Table 1 summarises the achievable front-end and synloop performances during a typical winter and summer day.
As can be noted during the winter day the FE load is kept constant (a fixed FE load is preliminarily selected for such a week), while the synloop load can move within the allowance (5%) provided as boundary conditions.
Being the green portion of the plant based on full PV power generation system, during daytime the hydrogen produced by the electrolysers is partially fed to the ammonia synthesis loop, while the balance is fed to hydrogen storage, this stored hydrogen will be used to enable the night operation of the green portion of the hybrid plant.
During a typical summer day, the FE capacity is kept constant and, as expected, at lower load compared with the typical winter day, because of the higher renewable power availability that would allow higher green hydrogen production from the electrolyser; during daytime this hydrogen is partially routed to the synthesis loop and partially to the hydrogen storage unit. During night-time stored green hydrogen is supplied to the synthesis loop.
From Table 1 it can be seen that:
- plant capacity is always kept between 97.5% and 102.5% compared to the baseline production;
- only the synloop must be able to change load quickly;
- summer balances are the furthest conditions from baseline (most green H2 + N2 input). Summer day-night transitions are therefore the largest jumps in operating conditions.
Turning the focus of the analysis to the ammonia synthesis converter, it can be easily assessed that Casale proprietary solutions for flexible Haber Bosch loops provide a constant synloop operating pressure as well as almost constant converter operating temperature. The purge gas in Table 2 was slightly changed however this is not a requirement of Casale’s proprietary solution for loop control.
As shown in Fig. 7 and for the selected example, the major factor affecting capex and opex, due to plant hybridisation is the cost of electricity and the electrolysers.
The hydrogen storage capex can be further reduced by allowing a final hourly production variation of higher than the assumed 5% (initial boundary conditions selected for the presented hybrid revamp example).
Conclusions
Fertilizer producers have a window of opportunity to adapt the operation of their existing plant as well as to develop new facilities to help reduce the disruption that climate change will ultimately bring.
New challenges towards sustainable power and chemical production to minimise the environmental impacts and carbon footprint are on-going and plant hybridisation of existing units provides significant advantages thanks to the availability of the existing plant sections, which minimise the capex investment required.
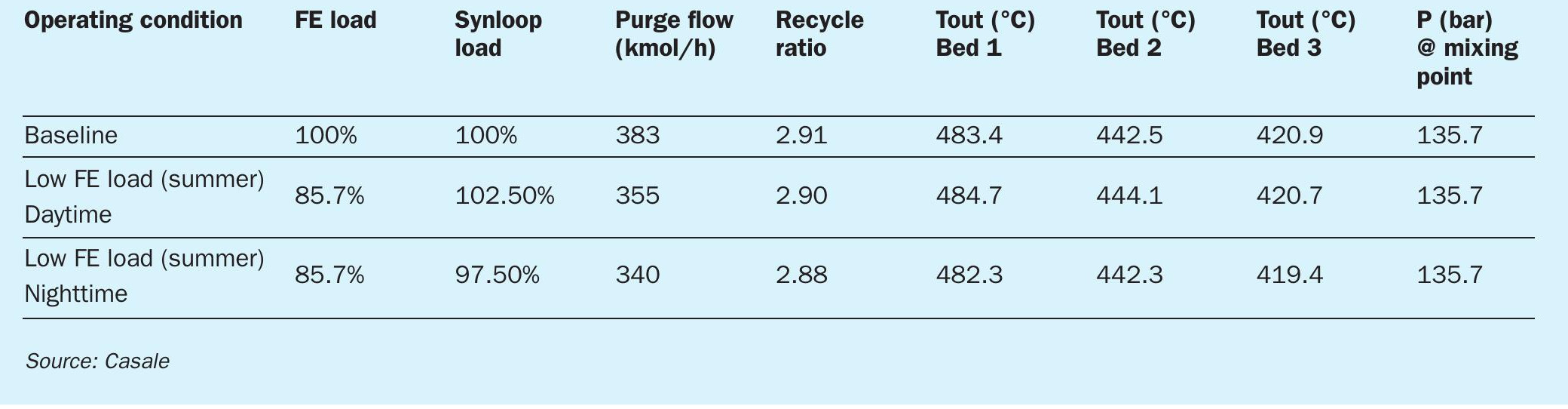
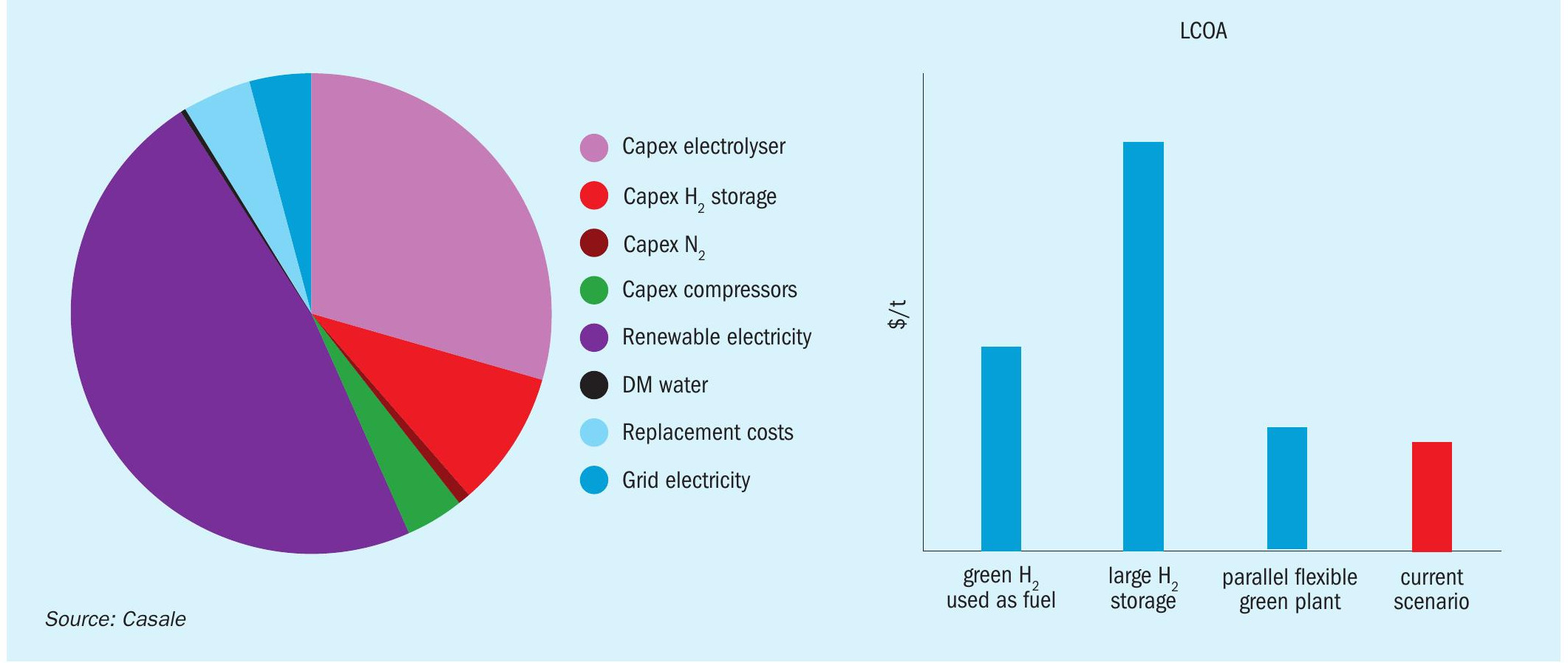
Casale has provided solutions focused on updating existing facilities by the integration of green hydrogen, in particular a new concept has been proposed for plant hybridisation involving erratic power supply without affecting the mechanical integrity of the existing plant.
The new proposed scheme provides an efficient arrangement targeted at reducing the carbon footprint and allows premium production in the most reliable and simple way.