Nitrogen+Syngas 387 Jan-Feb 2024
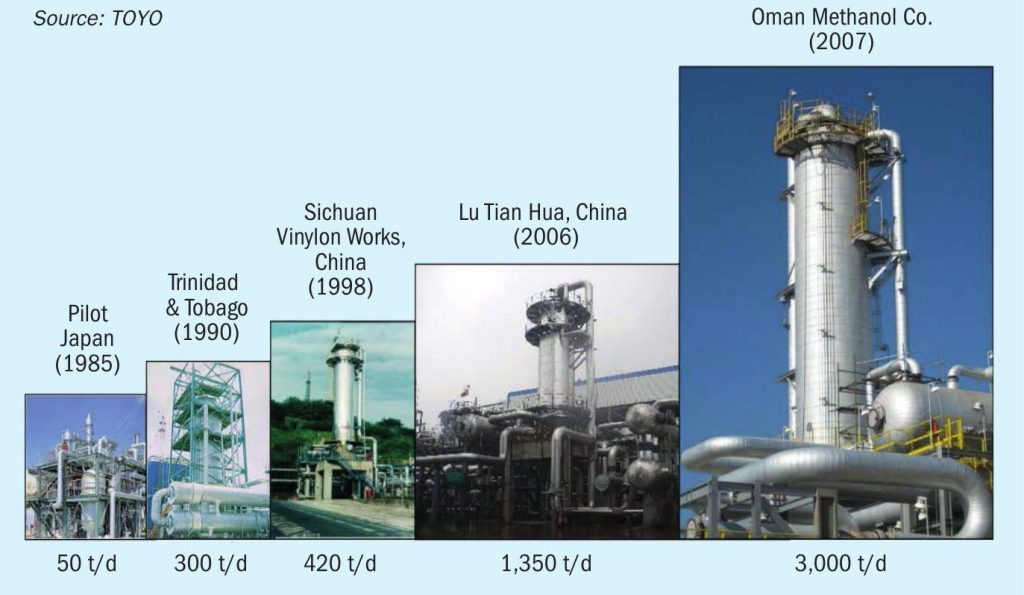
31 January 2024
Methanol synthesis using renewable energy
GREEN METHANOL
Methanol synthesis using renewable energy
Toyo Engineering Corporation (TOYO), a global leading engineering contractor, has developed g-Methanol® technology. Methanol, traditionally produced from fossil resources, is now gaining attention for synthesis using carbon dioxide and hydrogen from renewable sources. This article explores methanol synthesis technology and the challenges in utilising renewable energy sources.
To achieve carbon neutrality by 2050, there is a significant emphasis on capturing, utilising, and storing carbon dioxide (CO2 ). Methanol, traditionally derived from carbon-based resources like natural gas and coal, has been widely used as a chemical product and fuel. Recently, there’s been a shift towards methanol synthesis from CO2 and hydrogen from renewable sources. In Japan, methanol is attracting attention as a raw material for chemicals, mainly through ARPChem (Artificial Photosynthesis Chemical Process Technology Research Association) as well as e-fuel. This article explores methanol synthesis technology and the challenges related to using renewable energy.
Demand for methanol globally and in Japan
Methanol, consumed globally at 92 million tonnes annually, serves mostly as a raw material for chemicals (70%) and as fuel (30%)1 . Traditionally sourced from natural gas, recent focus has shifted to low-carbon alternatives like biomethanol and CO2 -derived methanol using renewable sources (Fig. 1). These variants maintain quality while reducing the carbon footprint of production. By using low-carbon methanol, existing applications can cut CO2 emissions without altering processes or facilities. Furthermore, in the fuel field, where CO2 emissions reduction is urgently needed, low-carbon methanol can be directly used as marine and automobile fuels. Additionally, fuels through processes such as MTG (Methanol to Gasoline) and MTJ (Methanol to Jet), can be deployed as automobile and aviation fuels that can be directly dropped into existing infrastructure without requiring a revamp.
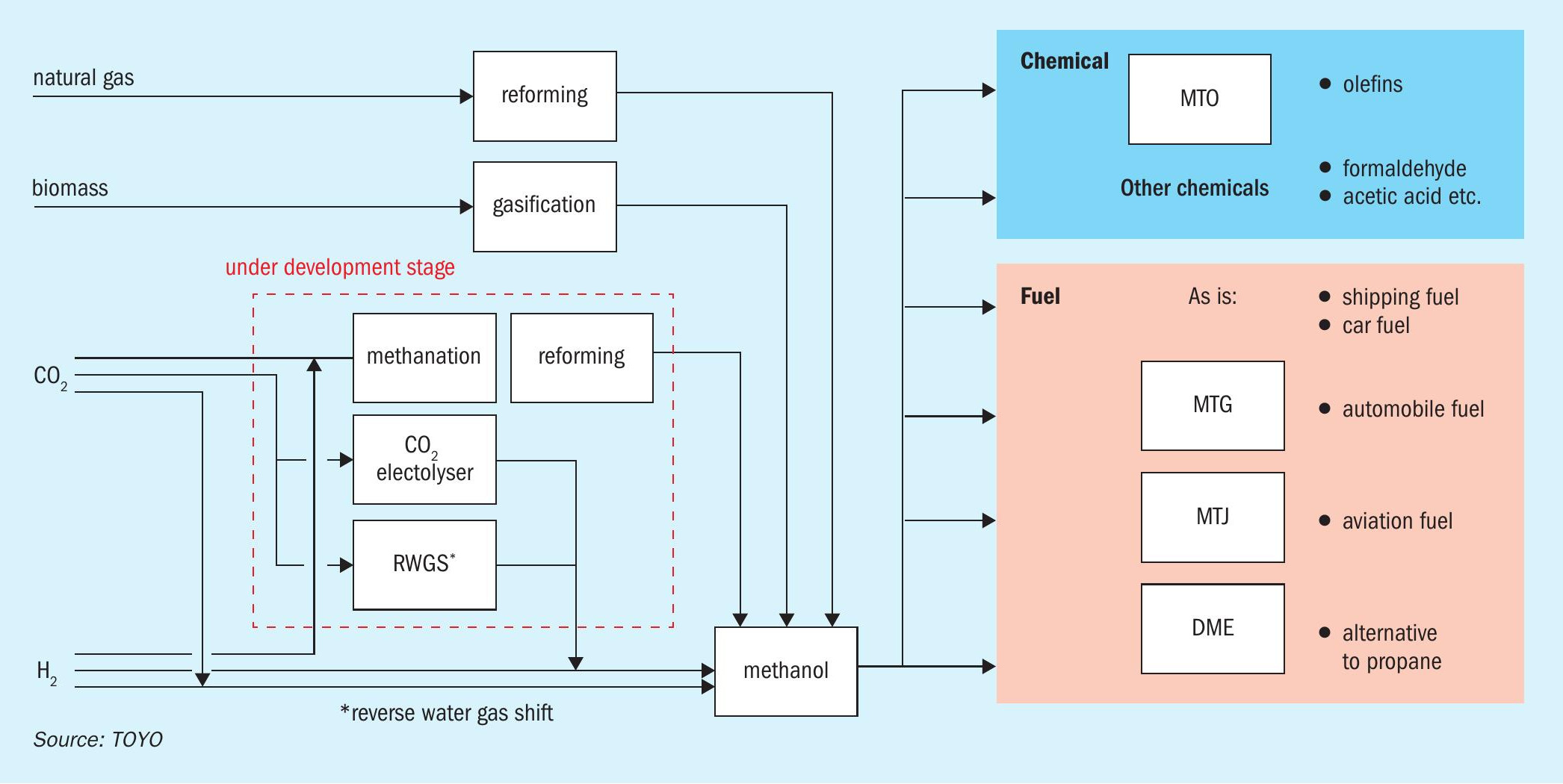
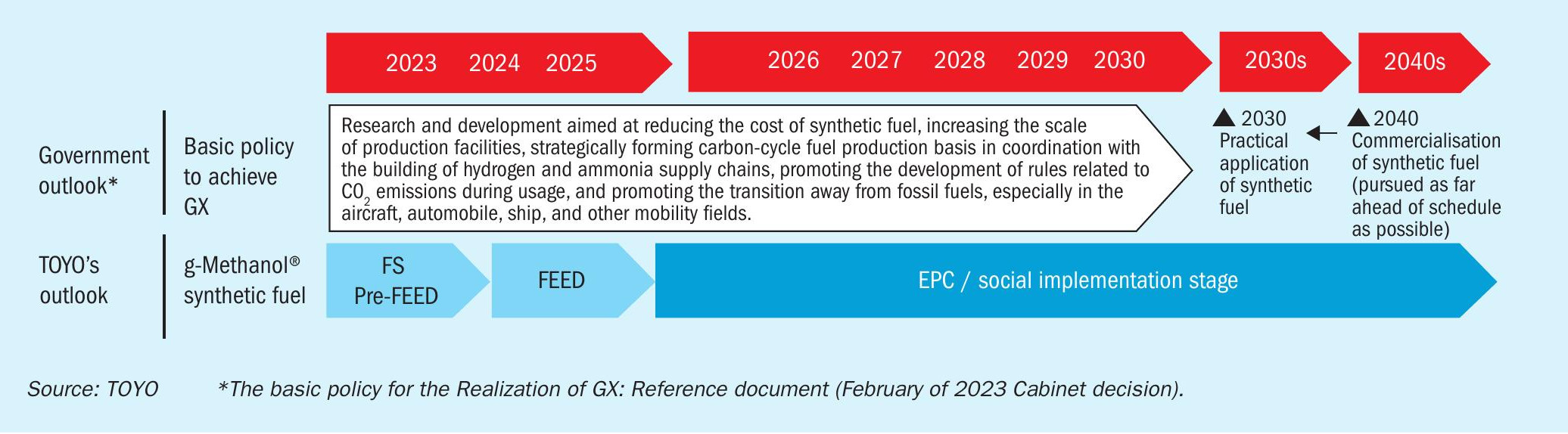
In the chemicals sector, olefins have so far been produced in China from coal-derived methanol by MTO (Methanol to Olefins). Low-carbon methanol could offer a clean route for olefin production. In addition to its conventional use, the promising expansion of methanol as a fuel is expected to rise dramatically to 500 million tonnes by 2050, marking a fivefold increase from 20202 .
In Japan, the carbon recycling roadmap was updated by Japan’s Ministry of Economy, Trade and Industry (METI). Methanol is highlighted as a key substance for chemicals and fuels. The national policy, announced in February 2023, outlines the future promotion of synthetic fuels as part of the GX realisation strategy, aiming to transition towards a carbon-neutral society using existing infrastructure and CO2 utilisation (Fig. 2). As hydrogen costs from renewable sources decrease, synthetic fuels are expected to gain traction. Japan targets commercialising these fuels by 2030 and aiming for widespread use by 2040, prioritising early adoption. In particular, methanol has already been commercialised as a marine fuel, and since the market can be shifted to aviation fuel in the future, CO2 methanol is expected to be implemented in society at a relatively early stage.
Methanol synthesis from CO2 and H2 from renewable sources
TOYO’s g-Methanol® process
TOYO is developing a low-carbon methanol process called g-Methanol® , utilising CO2 and hydrogen from renewable sources, as shown in Fig. 3. The hydrogen is generated through electrolysis using renewable energy.
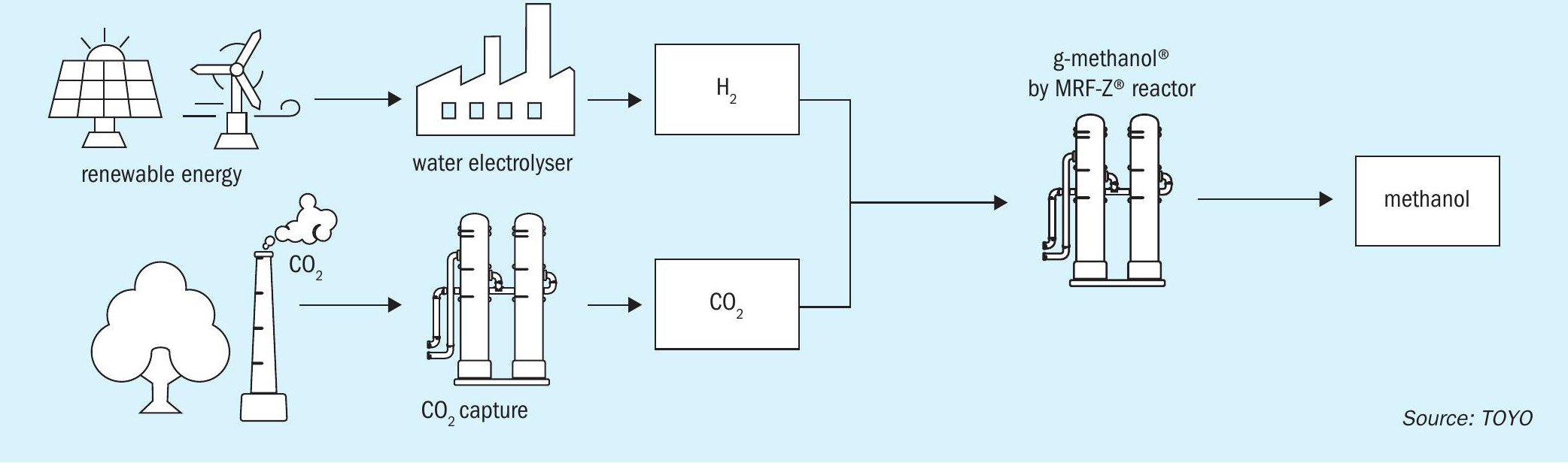
Fig. 4 shows a typical scheme for a CO2 methanol plant. The reforming process in the conventional natural gas scheme is replaced by a water electrolysis facility and a CO2 recovery facility, while the conventional schemes are applied to the synthesis and distillation sections. In terms of overall yield, the inputs are renewable energy, water, and CO2 , making it a low-carbon process compared to methanol derived from fossil resources.
Methanol synthesis reactor
Catalytic reactors in methanol plants play an important role in utilising their catalytic properties and improving process performance.
TOYO’s MRF-Z® reactor was initially demonstrated in Japan, with a 50 t/d pilot plant established in 1985. The largest production facility to date is 3,000 t/d. It is feasible to design a single system capable of handling 5,000 t/d class in this case for conventional methanol. The history of MRF-Z® reactor scale-up to date is shown in Fig. 5.
The MRF-Z® reactor recovers reaction heat as steam (Fig. 6). Synthesis gas enters from the top, spreads outward, then moves radially through the catalyst bed towards the central pipe (radial flow). Reaction-generated heat is recovered by circulating boiler water through cooling tubes in the catalyst bed, generating steam at saturation temperature and pressure. Control over catalyst bed temperature is achieved via steam pressure and inlet gas temperature.
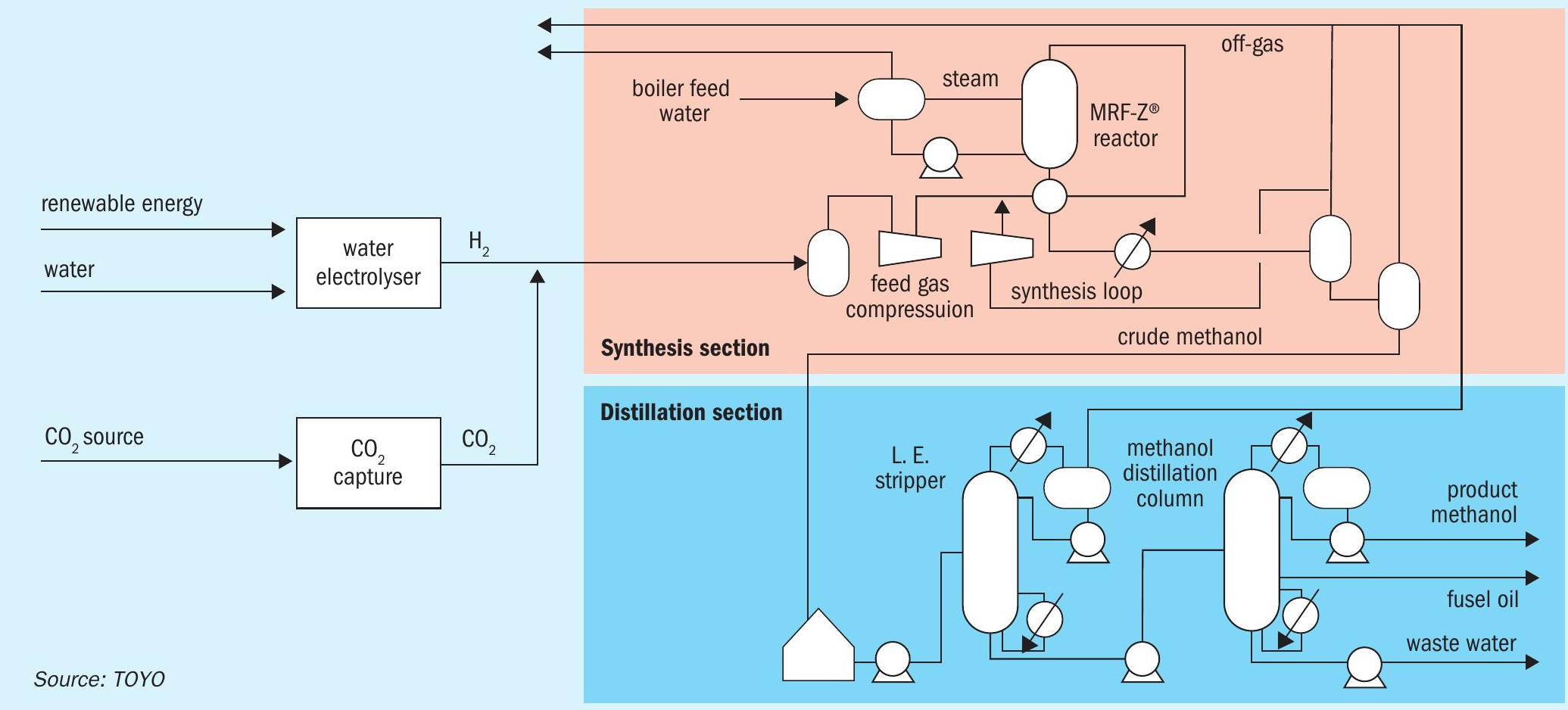
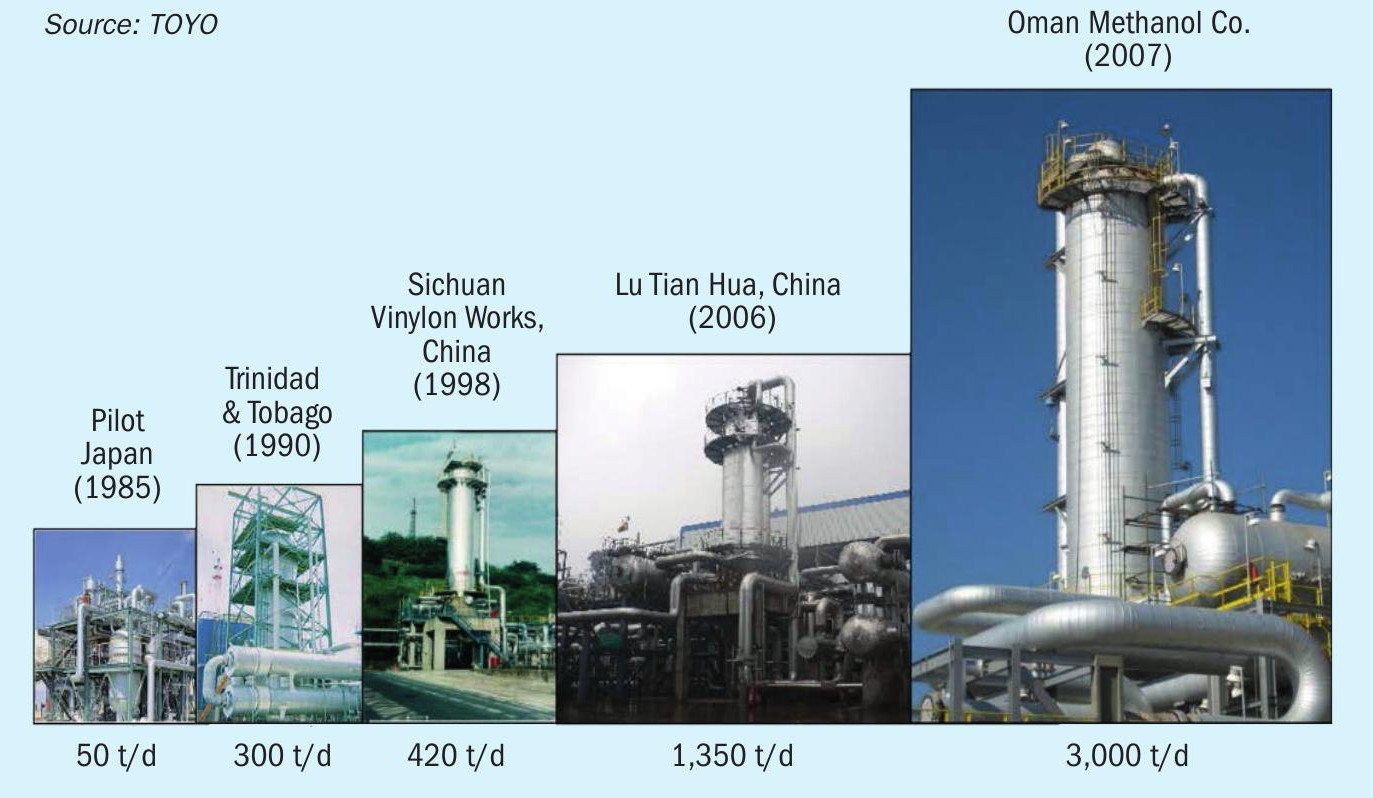
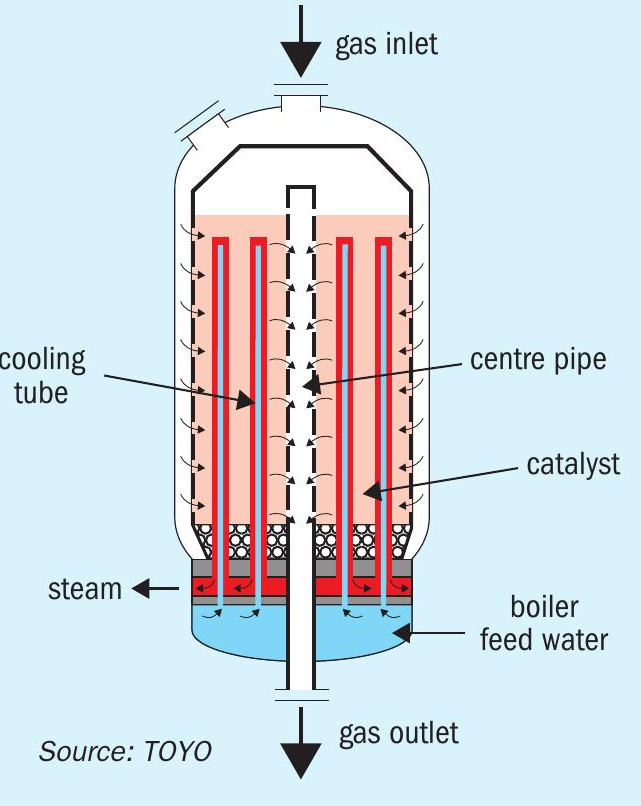
The MRF-Z® reactor offers the following advantages:
- The reaction heat generated by the methanol synthesis reaction can be recovered as medium-pressure steam through a cooling pipe and used in the process.
- By facilitating cross-flow of the gas over the cooling tube, a high heat transfer coefficient between the syngas and the cooling tube can be achieved, allowing for a reduction in the heat transfer surface area of the cooling tube.
- By arranging the cooling pipes at appropriate intervals, the reaction path in the methanol synthesis reactor can be placed on the maximum reaction rate line of the methanol synthesis reaction, maximising the methanol production per unit volume of catalyst. As a result, the size of the reactor can be reduced, making it easy to scale up (Fig. 7).
- Synthesis gas flows in a radial flow to reduce the pressure drop in the catalyst bed and thus reduce the power of the synthesis gas compressor.
- Simplified catalyst removal through the centre pipe saves time and manpower.
The MRF-Z® reactor suits exothermic methanol synthesis due to its adaptable surface area and temperature control. Its features align well with current catalyst limitations, expected to have shorter lifetime due to by-product water, necessitating more frequent replacements.
Challenges when using H2 from renewable energy sources
In conventional methanol production plants from fossil resources, a stable supply of raw materials allows for relatively straightforward continuous operation. In contrast, when generating hydrogen using renewable energy, the flow of hydrogen needed for methanol production fluctuates with variations in electricity generation due to day-night cycles and weather conditions. To ensure a consistent supply of renewable energy, the ideal approach involves purchasing grid power with green certificates or combining multiple renewable energy sources such as solar, wind, and pumped storage. However, practical implementation faces challenges on both regulatory and cost fronts. TOYO has been working on developing a system that is flexible enough to cope with fluctuations in the amount of electricity generated from renewable energy sources.
TOYO has developed MethaMaster™ software to optimise the configuration of plant equipment based on the power generation profiles of each plant (Fig. 8). This software enables simulation of the plant’s average production, operating rate, shutdown frequency, etc., by inputting TOYO’s g-Methanol® process performance, cost information of each facility, etc., in addition to the power generation change profile. Based on the results, the plant configuration and capacity of each facility are considered, and an optimised average methanol production cost, LCOM (levelised cost of methanol), is determined.
Compared to a system with a plant receiving stable power, additional facilities are needed:
- During periods when renewable energy is sufficiently supplied, facilities for storing electricity through batteries or producing and storing hydrogen are employed.
- During periods of insufficient renewable energy, such as during the night, power generation facilities like hydrogen gas turbines are utilised to continue operations at low loads.
- Fig. 7: Cooling tube arrangement and maximum reaction rate line4
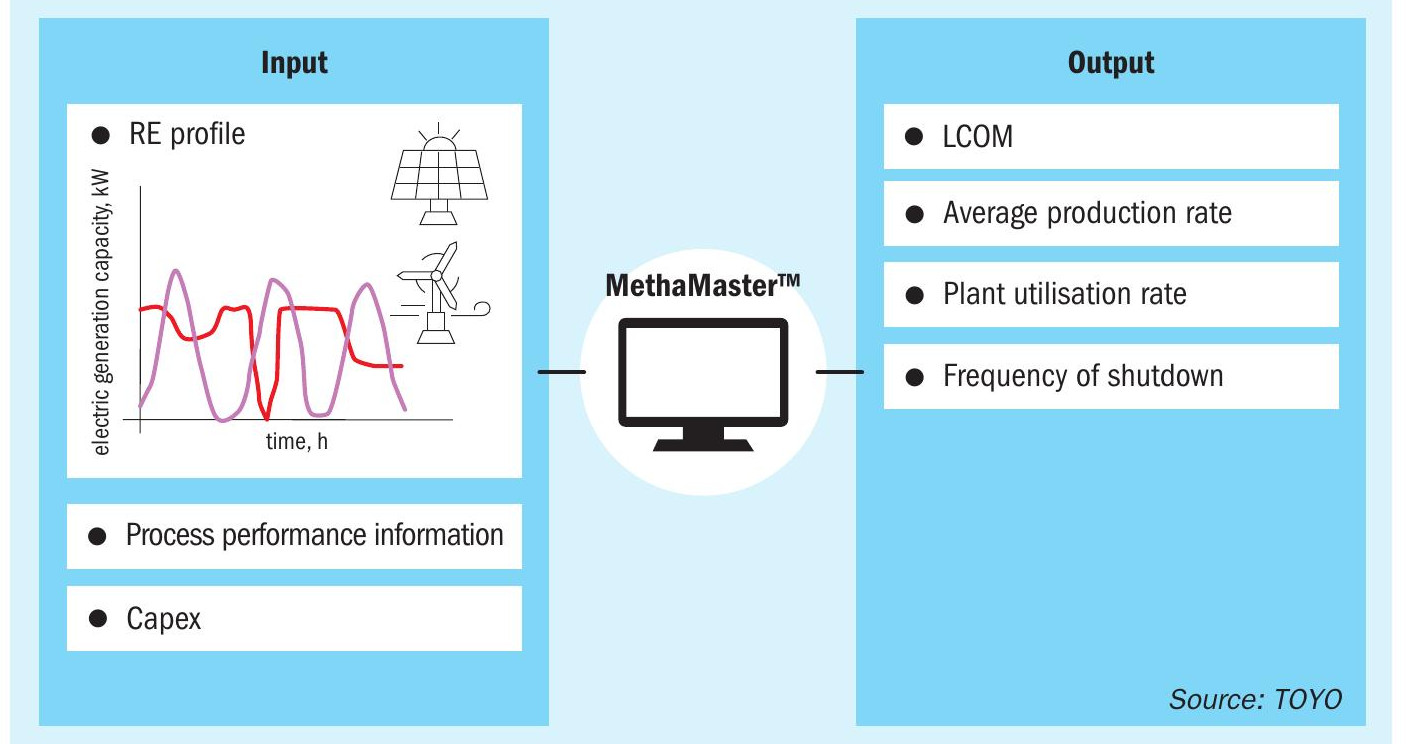
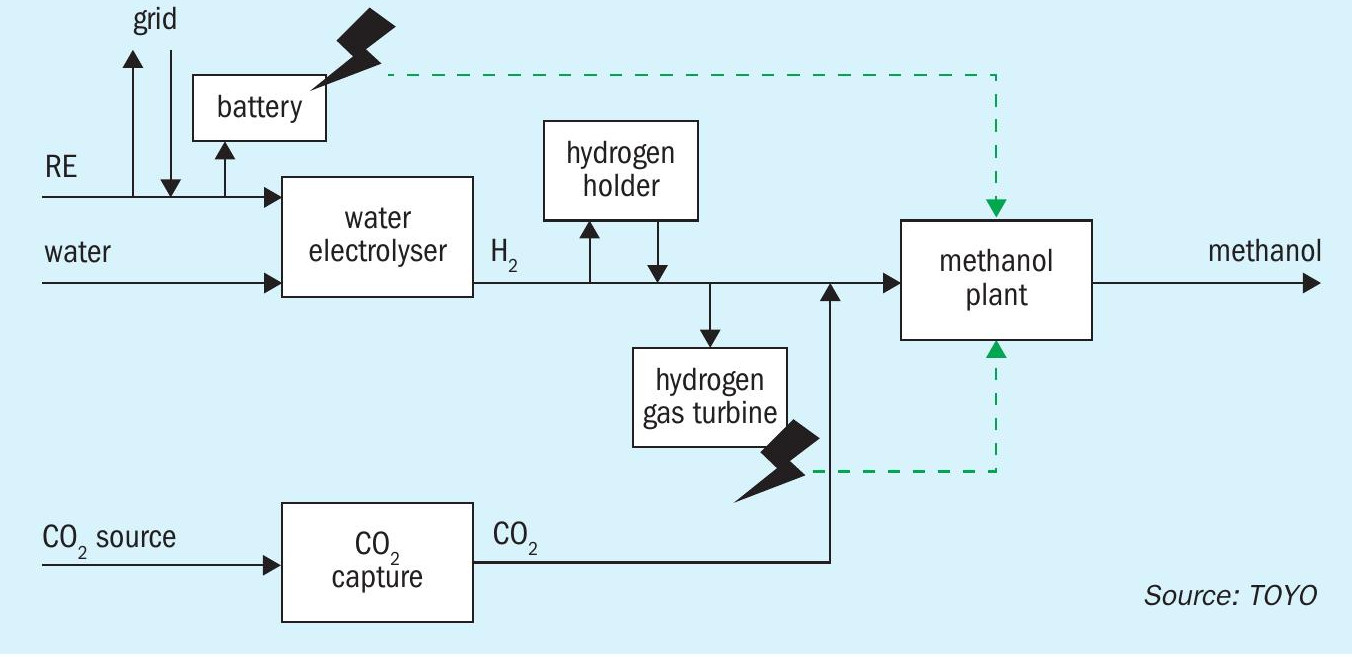
Fig. 9 shows an example of this plant configuration.
Integrating renewable energy complicates facility capacity planning. Unlike traditional plants aligned with steady supply and demand, renewable energy introduces factors like variable power generation patterns and the methanol plant’s flexibility in managing load changes. Capacity decisions must now consider both demand and the specifics of renewable energy and methanol synthesis processes to handle operational fluctuations.
Conclusion
This article focuses on low-carbon methanol production technology using renewable energy, exploring how fluctuations in power generation affect plant design and production costs. Methanol synthesised from CO2 and hydrogen from renewable sources holds significant promise in achieving a carbon-neutral society and early adoption of synthetic fuels. Its capacity to handle substantial CO2 volumes, technological maturity, and versatility across various markets underscore its potential.
References