Sulphur 396 Sept-Oct 2021
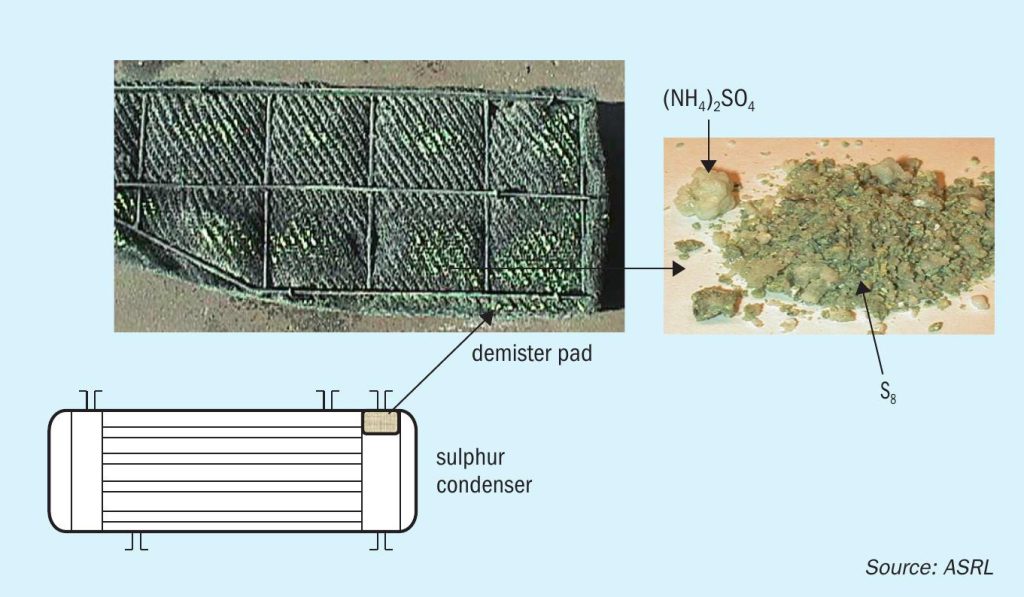
30 September 2021
Ammonium salt formation conditions based on measured vapour pressures
CLAUS SULPHUR RECOVERY UNIT
Ammonium salt formation conditions based on measured vapour pressures
ASRL has conducted studies on ammonia destruction in the sulphur recovery unit (SRU) for over a decade1-5 . Other studies at ASRL have investigated mechanisms for ammonium salt formation and deposition downstream in the Claus plant, as well as the potential sources of ammonia (NH3 )in a gas plant7 . A less understood subject is addressing how much residual NH3 is tolerable or at what temperature will residual NH3 cause ammonium salt deposition. In this study, existing knowledge on thermal stability of ammonium salts and new measurements have been used to identify the gaseous components required for deposition, through reversible vapour pressure expressions.
Ammonium sulphateis ((NH4)2SO4) very stable and requires only small amounts of SO3 to lead to deposition, even with small amounts of NH3 . A potential source of SO3 is a malfunctioning acid gas reheater or a natural gas reheater (excess O2 or poor mixing) leading to “lead chamber cycle” chemistry involving NO26 . Other gaseous species could lead to the formation of ammonium salts, albeit at cooler temperatures than anticipated. With this knowledge, this work sought to determine the levels of ammonia necessary for the formation of various ammonium salts and further study the gaseous species in equilibrium with some common salts.
As expected, measured vapour pressure data showed that ammonium sulphate vapour pressure was very low, suggesting that the salt can easily be formed below 250°C and is quite stable. Again, the stability of ammonium sulphate means that very low concentrations of ammonia can lead to direct precipitation if any SO3 is present. Ammonium sulphite on the other hand was found to be very unstable over 70°C, which suggests that it will not form in Claus conditions. Ammonium thiosulphate (NH4 )2 S2O3 ) was found to be the most likely species to be formed in the absence of SO3 and below 150°C based on the measured vapour pressures. Note that subsequent formation of ammonium sulphate proceeds through the reaction between the H2O and thiosulphate, thus discovery of ammonium thiosulphate or ammonium sulphate in or downstream of an SRU can be evidence for initial thiosulphate deposition from SO2 , H2O and NH3 . Here the thiosulphate requires cold temperatures for initiation (83 to 98°C).
Ammonium salt deposition in Claus plants
In a refinery, ammonia will come from the hydrotreaters and is often sent with H2 S (sour water stripper gas) to the front end of the thermal reactor in a Claus plant. Claus plants designed for ammonia destruction convert the majority of the ammonia to N2 . Incomplete ammonia destruction is thought to lead to deposition of ammonium salts downstream of the thermal reactor in the SRU; however, how much residual NH3 is required to cause issues is often not clear. Furthermore, it’s not just NH3 that leads to a salt issue. Some SRUs seem to operate successfully with 150 ppmv of NH3 while others do not.
As expected, the colder surfaces are where salts will deposit, thus evidence of deposition has been typically seen on the demister pads in the last condenser and in lines leading to the thermal oxidiser (Fig. 1). In a gas plant, sources of ammonia are not well understood, and suggestions have included the amine degradation in gas sweetening units or amine carry over to the Claus plant. ASRL has done some work to show that rates of ammonia formation in amine units are too low to account for ammonium salts found in some gas plants7 .
Ammonium salt formation in Claus plants is difficult to predict given the fact that ammonia levels ranging from 40 to more than 150 ppm have been detected in some gas plants (both DEA and MDEA systems) with no deposition issues; however, some plants experience ammonium salt deposition. The question then remains how much ammonia causes issues with salt deposition and what is the mechanism of formation of these salts (what other species are required). Even more important is the question of whether one can rely on accurate salt vapour pressure to predict the possibility of ammonium salt deposition in a Claus environment.
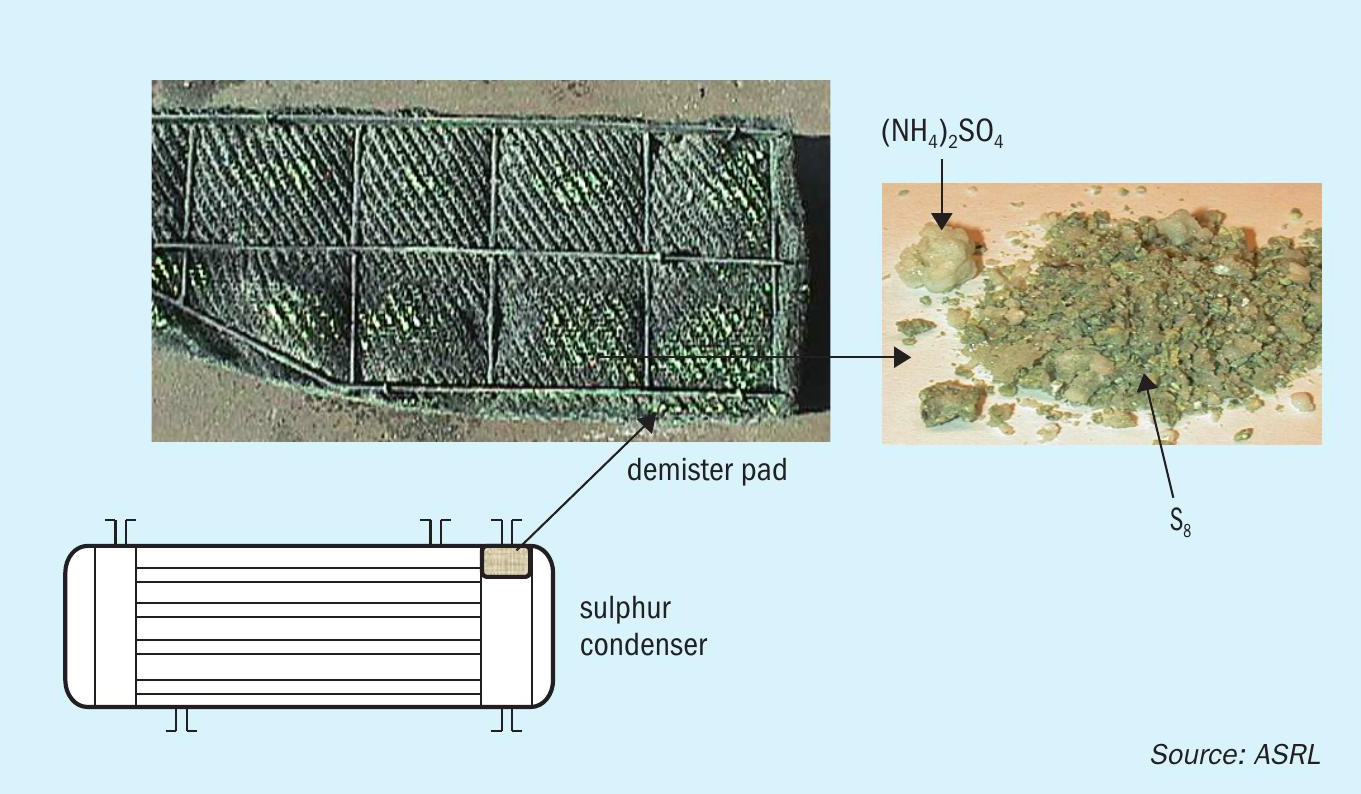
In order to examine the potential deposition of ammonium sulphate, it is also important to consider the sulphuric acid dew point in a Claus plant 8,9 . Sulphuric acid can be the precursor acid for the formation of ammonium sulphate. The vapour pressure indicates that ~1 ppm SO3 would cause acid liquid to form at 130°C. Note that SO3 is not stable under the thermal reactor temperatures, in particular under reducing conditions in the Claus reactor 6 . Sulphuric acid vapour pressure is greater than the vapour pressure of the ammonium sulphate salt; therefore, sulphuric acid is not considered a condensable product in the presence of ammonia although it could be an intermediate material.
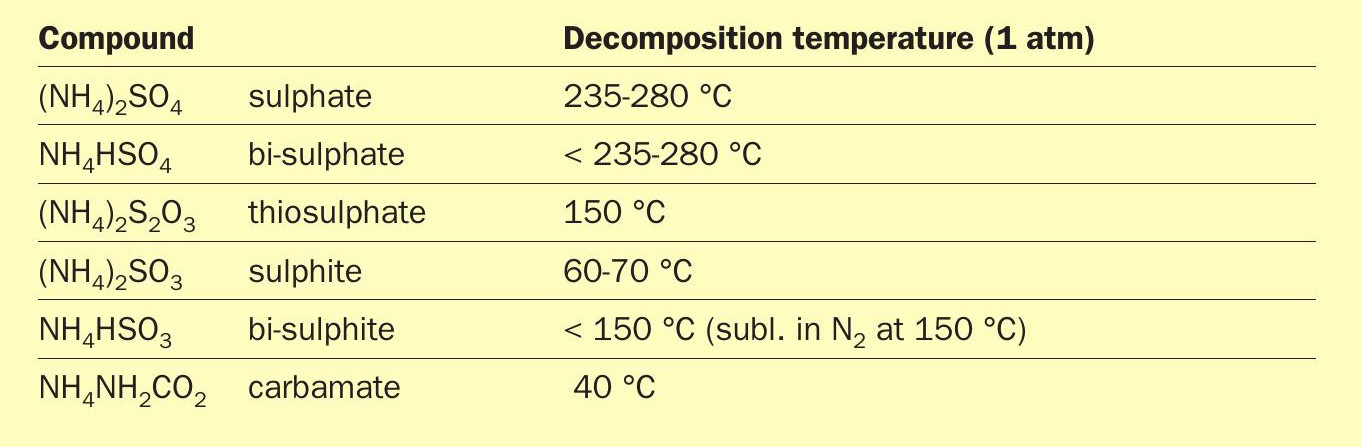
Existing literature data on the thermal stability of ammonium oxy-sulphur compounds at atmospheric pressure (Table 1) shows that ammonium sulphate is the most stable salt below 250°C 1,10-12 . It also suggests that this would be the most stable salt to be deposited downstream of the thermal reactor in a sulphur plant. The mechanism for the formation of the ammonium sulphate and what levels of ammonia would be sufficient to cause deposition are poorly defined, because of the scarcity of the counter ion.
Ammonium salt deposition through a mechanism involving both the sulphate and thiosulphate species as part of the H2S/SO2 chemistry in the Claus process in both the gas phase and catalytic converters was identified by Clark and others (2006). The same study also demonstrated that SO3 formation is not a prerequisite for ammonium salt deposition at the cooler temperatures. Note that several projects at ASRL have demonstrated the formation of ammonium thiosulphate as the most prevalent species below 230°C (without SO3 in the system). This is not to say that ammonium sulphate would not deposit directly if SO3 were available after the waste heat boiler, so we need to pay attention to both mechanisms.
The equilibrium decomposition of ammonium sulphate was investigated by Kiyoura and Urano (1970), using differential thermal analysis (DTA), thermogravimetric analysis (TGA), chemical analysis and X-ray diffraction technique, from 140°C to 240°C 13 . Several possible intermediate species were identified and several steps proposed for the decomposition. The two-step decomposition is given in Reaction 1 with the overall equilibrium expression given by Equation 2.
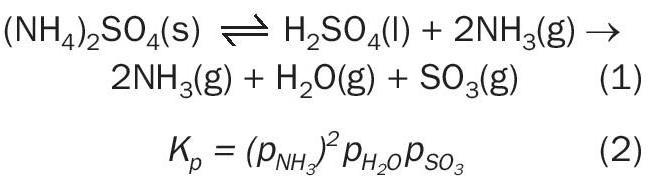
Ammonium sulphite decomposition equilibrium was investigated by Scargill (1971), utilising chemical analysis and IR spectroscopy to identify chemical species while the dissociation equilibrium was derived from the transpiration method. Reaction 3 shows the reversible decomposition for the anhydrous salt. The equilibria shown by Scargill (1971), was based on experimental measurements from 0 to 23°C: 14
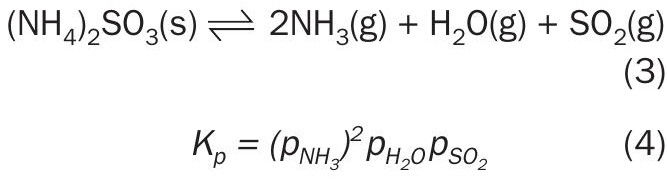
The ammonium thiosulphate decomposition reaction can be expressed as a two-step process shown as Reactions 5 and 6. This work seeks to verify the equilibrium species that exist in detectable quantities for this system based on mass spectrometric analysis and quantification of condensed sulphur left behind by CS 2 solubility.
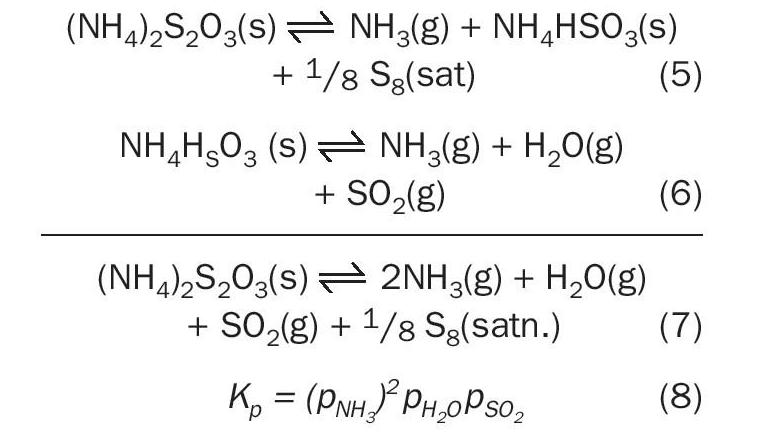
With previous knowledge about the thermal stability of the various ammonium salts, this work was designed to determine the lower limits (temperature and concentrations) over which the salts can deposit and the gaseous species involved in the equilibria. The experimental approach taken was to measure vapour pressure data that can be used to estimate deposition conditions. The use of cold trapping techniques to measure vapour pressure have been used in literature and it was also employed in this work. Its accuracy relies on the efficiency of the trapping media and how well the deposited salt can be recovered and analysed. It is limited by the fact that a few components can be analysed. Results obtained from the trapping technique for ammonium sulphate were not very accurate compared to available literature data. GC and MS techniques gave more accurate results which are presented in this work.
Ammonium sulphate vapour pressure and deposition
Literature data gives a wide decomposition temperature range for ammonium sulphate between 235-280°C (Table 1). Accurate values of the equilibrium constants for the reversible decomposition of the salt would be useful to calculate vapour pressure at any given set of temperature and pressure. In ASRL’s work, decomposition of ammonium sulphate (Reaction 1) was measured at T = 200°C to 250°C using direct injection GC-TCD.
The experimental data together with literature data 13 were used to fit an expression for the equilibrium constant (KC), Equation 9 (T is in Kelvin):

Fig. 2 is a graphical representation of the ammonium sulphate equilibrium as a function of temperature which shows that the data obtained in this work is in good agreement with existing literature data. The results presented in Fig. 2 illustrate that the ammonium sulphate equilibrium constant is very small. Based on the sulphuric acid dew point calculations, it would mean that under normal operating conditions, very low levels of ammonia and SO3 would lead to ammonium sulphate deposition in all three converters before the condensation of sulphuric acid. For an example calculation, according to this low vapour pressure, 150 ppm NH3 and 0.40 atm H2O at T = 130°C would lead to formation of solid ammonium sulphate with just 3×10 -19 atm SO3 . In another example, 150 ppm NH3 , 1 ppb SO3 and 40% H2O shows a solid formation temperature (saturation) at T = 191.4 °C.
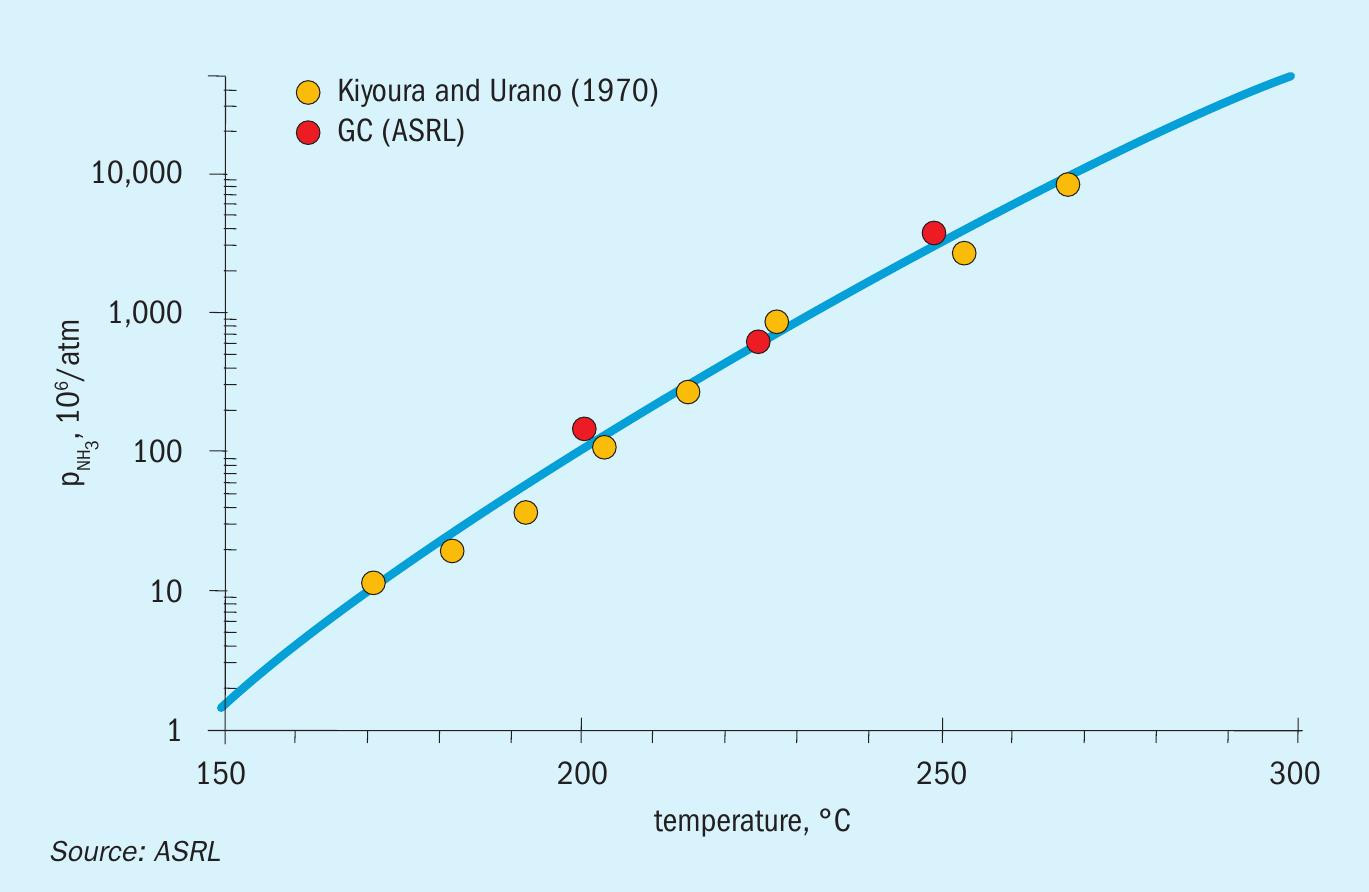
This suggests that deposition will be an issue with low levels of ammonia if SO3 is added to the SRU. Again, this can come about through direct fired reheaters which are operating with enough O2 to generate SO 3 or with poor mixing (lead chamber chemistry). Note that this implies that the ammonium sulphate formation does not have to involve the condensation of sulphuric acid or SO4 , although the acid may be an intermediate species. This is particularly true if any sulphurous acid is condensed, where disproportionation can lead to small amounts of sulphate.
Ammonium sulphite vapour pressure
Decomposition of ammonium sulphite was measured using GC-TCD. Ammonium sulphite was not stable above 70°C. Tests were conducted on the hydrated salt (NH 4 ) 2 SO 3 .H 2 O , for which the decomposition was assumed to follow Reaction 10.

The corresponding equilibrium equation determined from ASRL results and literature data (Scargill, 1971) 14 is:

Results obtained in this work agree with literature data within experimental uncertainty 14 . This is consistent with the low decomposition temperature and ambient pressure reported in literature. The equilibrium constant for ammonium sulphite shows that low levels of ammonia could deposit ammonium sulphite below 60°C; however, this is below the sulphurous acid and/or water dew point in the system. Thus, ammonium sulphite is not likely to be a depositable species in the SRU.
Ammonium thiosulphate vapour pressure
The decomposition of ammonium thiosulphate was followed using mass spectrometry from 60°C to 120°C while analysing all gaseous components. The species analysed included SO3 , H2S, SO2 , NH3 and H2O and S8 . Fig. 3a shows MS results plotted as raw detector counts, with increasing levels of H2O, NH3 and SO2 from 70°C to 120°C. The small deviation from the theoretical SO2 :H2O ratio of 1:1 is because of the use of raw mass counts on the graph. The average ratio of SO2 :NH3 from measured gas concentration above 80°C was 2.05 ± 0.02. In order to show what is happening below 70°C, Fig. 3a has been replotted as Fig. 3b with a logarithmic axis to focus on the low intensity peaks. A low signal-to-noise ratio below 200 minutes (T < 90°C) was observed. Also evident from Fig. 3b is evidence of low levels of H2S, which increase up to 120°C. There was no measurable level of SO3 in the system. There was visual evidence of S8 build up (seen as a yellow colour) on the ammonium thiosulphate bed. Formation of significant levels of SO2 , NH3 and H2O and S8 supports the applicability of Reaction 7 as the reversible decomposition or formation reaction for ammonium thiosulphate. The corresponding calibrated equilibrium expression for reversible decomposition of ammonium thiosulphate is given by Equation 12.

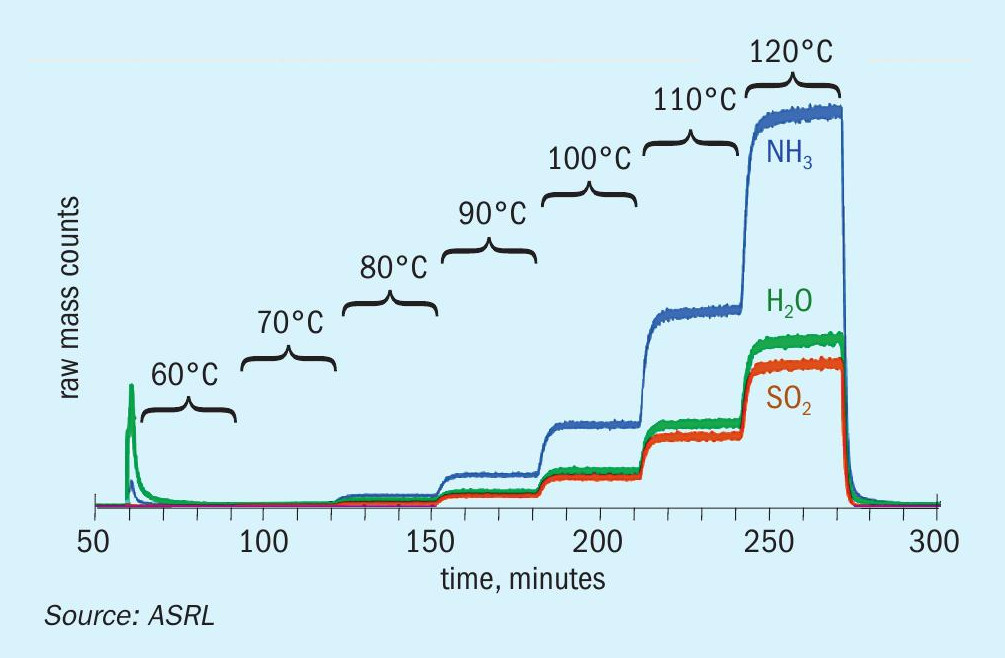
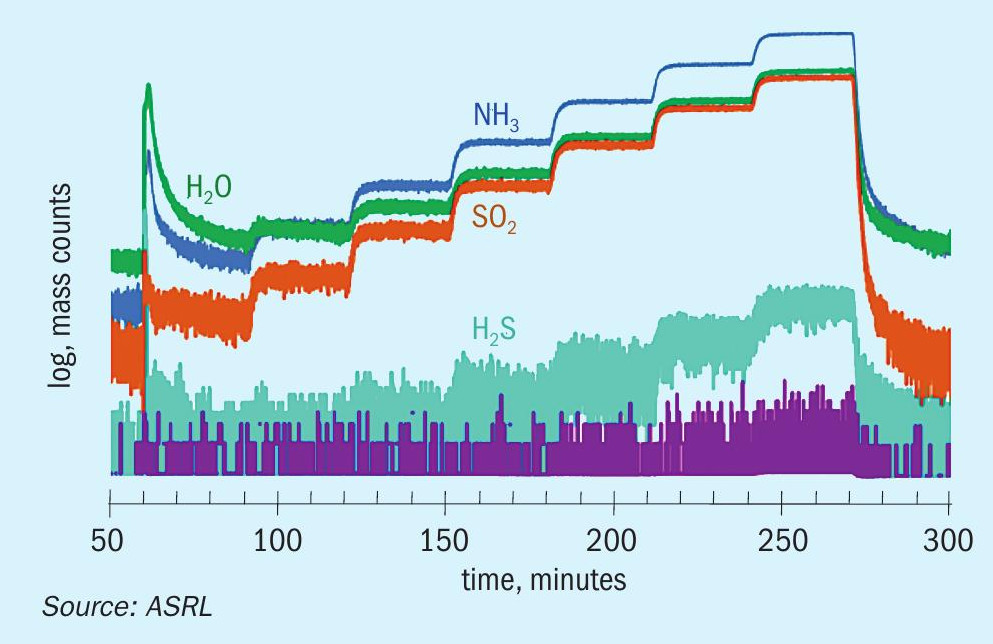
Only experimental data above 90°C (two independent sets of data) were used to calibrate Equation 12. The results together with calibrated equation are graphically presented in Fig. 4. The thiosulphate equilibria shown in Fig. 4 were highly reproducible as shown by both the duplicate set of results for ammonia vapour pressure as well as the results derived from the SO2 measurements.
By considering the presented vapour pressure data, ammonium thiosulphate is the most likely salt to be formed in cold sections of the SRU when SO 3 is not present. Before exploring some deposition conditions, ASRL wanted to verify that other species in the SRU would not influence this equilibrium. Additional experiments were conducted to explore the equilibrium shift on addition of different species to the thiosulphate system. Low levels of H2O, H2S, SO2 and NH3 were added to the system as individual gas mixtures balanced with nitrogen. The H2O, SO2 and NH3 additions shifted the equilibrium in line with Le Chatelier’s principle of disturbing a dynamic equilibrium. Both H2O, SO2 lowered NH3 partial pressure and NH3 addition lowered SO2 partial pressure. Addition of H2S proceeded to generate more sulphur from the Claus reaction with SO2 . Fig. 5 shows the decreased SO2 signal on addition of H2S to the system, as well as an increase in the H2O level consistent with the Claus reaction.
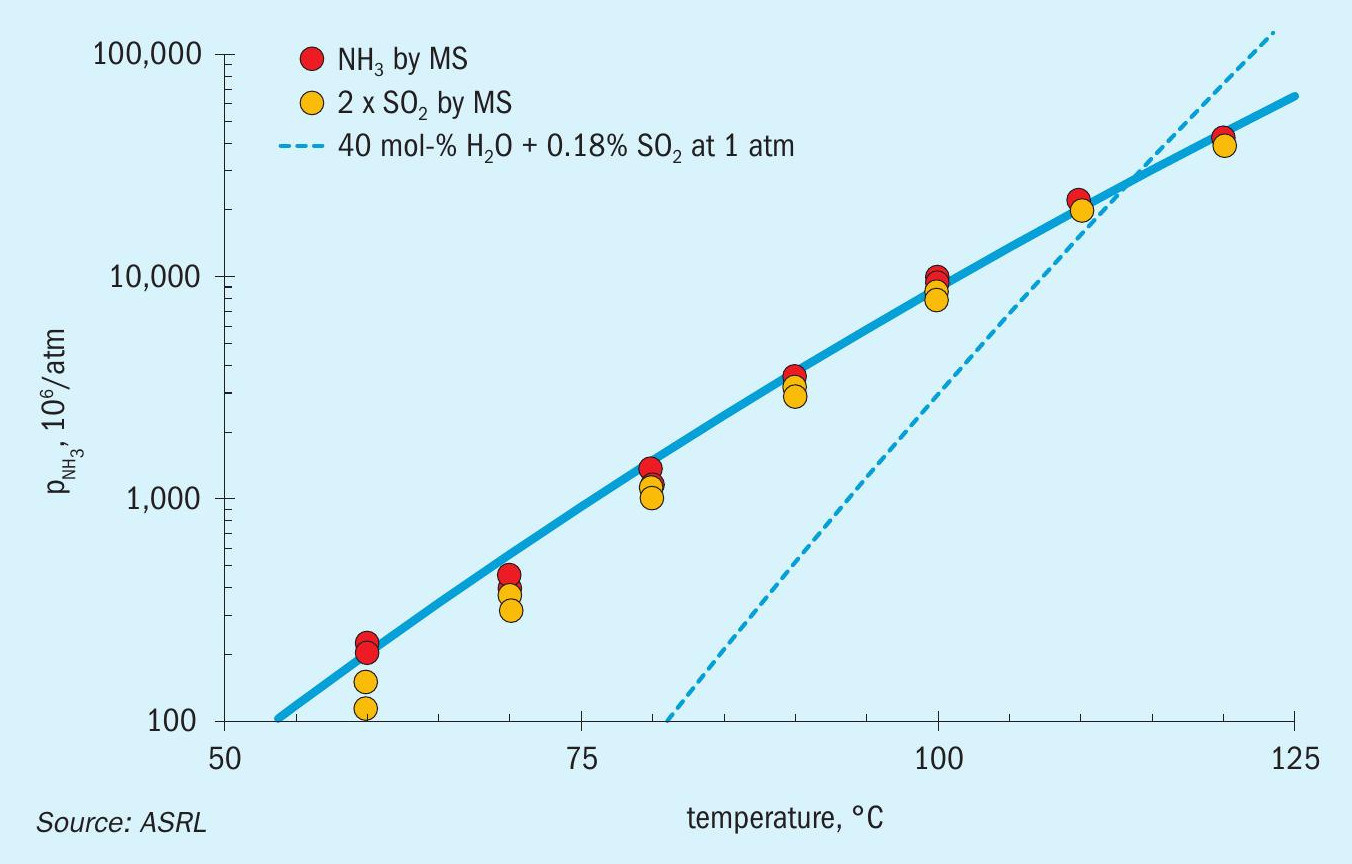
Separate experiments conducted at 120°C while flowing N2 over the solid, were conducted to verify the thiosulphate decomposition reaction through the S8 mass balance. The mass of sulphur collected at the end of test was within 97% of that obtained from the measured moles of SO2 . This supports the ratio for 1 SO2 : 1/8S8 in Reaction 7.
Note that ammonium thiosulphate reacts with H 2 O to form the more stable sulphate salt:

In other furnace studies carried out at ASRL, with partial destruction of NH3 and intentional trapping at T > 80°C, significant amounts of ammonium thiosulphate salts have been found. After some time, these salts can show up as a mixture of the thiosulphate and sulphate salt. Thus, finding ammonium sulphate in a SRU could be due to either ammonium thiosulphate deposition, followed by sulphate formation, or direct ammonium sulphate formation in the presence of SO3 . Note that a transient event, leading to a finite amount of either salt, could lead to an unexpected trapping of liquid water and/or higher insulating behaviour for internal surfaces.
Thus far, equilibria expressions 9, 11 and 12 have been presented for calculating solid formation temperatures or maximum concentrations for ammonium sulphate, sulphite and thiosulphate. Where most simulations rely on Gibbs Energy Minimisation (GEM) routines for complex equilibria in the SRU, ASRL integrated Equations 9 and 12 into the gas phase GEM simulations which also incorporate gaseous sulphuric acid. This allows to solidification to be explored under various Claus conditions.
ASRL explored several configurations using a 90/10 acid gas + sour water stripper gas [1:1] and 150 ppm of undestroyed NH3 . For a standard three-stage Claus at a 2:1 ratio, ASRL calculated a solid ammonium thiosulphate salt formation temperature at 84°C. This formation temperature increased to 89°C with 100% oxygen enrichment. All of these temperatures are below the surface temperatures within a well designed and well operating sulphur plant. The one exception could be the final flow line to the thermal oxidiser, where salt deposition has been noted in the past. Note that an 8:1 ratio dropped the solid formation temperature by 20°C.
It is possible that under an upset condition, the SRU may see 2% SO2 . In this case, the formation temperature with 150 ppm NH3 would be 90°C. Finally, if under upset condition, the total SRU pressure increases to 2 atm, then the salt formation temperature would be 98°C. All of these scenarios require quite low temperatures.
Finally, ASRL explored how much NH3 could be tolerated at 2% SO2 and 40% H2O and reasonable operating cold temperature of 130°C. In this case 10% (100,000 ppm) NH3 would be required for deposition. In other words, if there is no SO3 , eliminating cold spots should mitigate ammonium salt deposition. Based on these exploratory numbers, one can expect that the majority of salt deposition issues are caused by SO3 forming ammonium sulphate due to malfunctioning fired reheaters; however, deposition in very cold spots can still be initiated with just SO2 and H2O at 84 to 98°C.
Conclusions
Where ammonia is not completely destroyed in the front-end thermal reactor of a Claus plant, residual NH3 can lead to ammonium salt deposition under the right conditions. In order to gain a better understanding of appropriate deposition conditions, vapour pressure compositions have been measured for pure ammonium sulphate [(NH4 )2 SO4 ], sulphite [(NH4 )2 SO3 ] and thiosulphate [(NH4 )2 S2 O3 ]. From these measurements, vapour pressure expressions have been presented for exploratory calculations and incorporation into ASRL’s GEM simulation routines. ASRL’s study has found that virtually any SO3 in combination with any amount of NH4 will thermodynamically result in direct formation of ammonium sulphate salt. If SO3 is not present, then ammonium sulphate can form by first solidifying ammonium thiosulphate on surfaces at 84 to 98°C, given 150 ppm NH3 and various quantities of SO2 . Ignoring deposition kinetics, ammonium thiosulphate at these temperatures would also coprecipitate with sulphur. Thus, either significantly cold surfaces or the introduction of SO3 can result in ammonium salt formation. At 130°C, ASRL experiments suggest that SO3 would be needed to form ammonium salt in the SRU.
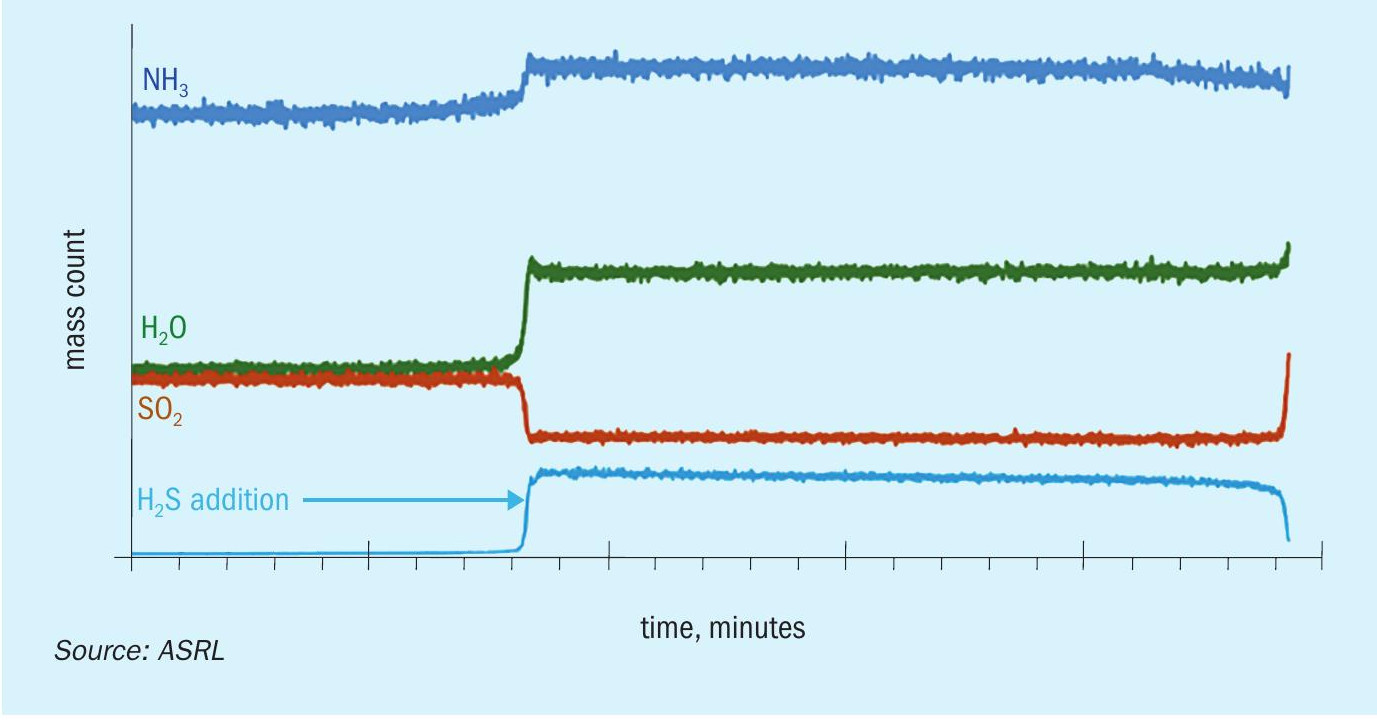
Well designed and robust front end thermal reactors, acid gas reheaters and fuel gas reheaters should avoid the production of SO3 through lead chamber chemistry. If faced with ammonium salt deposition, elimination of cold spots should coincide with the elimination of any source of SO3 . Future work could be aimed at investigating the fate of SO3 after passing through the Claus converters, where it is not known if all SO3 would survive these beds.
References